Freshwater acidification
Freshwater acidification occurs when acidic inputs enter a body of fresh water through the weathering of rocks, invasion of acidifying gas (e.g. carbon dioxide), or by the reduction of acid anions, like sulfate and nitrate within a lake.[1] Freshwater acidification is primarily caused by sulfur oxides (SOx) and nitrogen oxides (NOx) entering the water from atmospheric depositions and soil leaching.[1] Carbonic acid and dissolved carbon dioxide can also enter freshwaters, in a similar manner associated with runoff, through carbon dioxide-rich soils.[1] Runoff that contains these compounds may incorporate acidifying hydrogen ions and inorganic aluminum, which can be toxic to marine organisms.[1] Acid rain is also a contributor to freshwater acidification. It is created when SOx and NOx react with water, oxygen, and other oxidants within the clouds.[2]

What contributes to freshwater acidification?
The buffering capacity of soils and bedrocks within the freshwater ecosystem can contribute to the acidity of the water.[1] Each freshwater reservoir has a capacity to resist changes in pH, but an excess input of acids into the reservoir can cause the buffering capacity to decrease, eventually causing the water to become more acidic.[1] An increase in atmospheric CO2 affects freshwater acidity as the more of it dissolves into the water, the more acidic it becomes.[3] It is difficult to quantify the effects of anthropogenic CO2 due to the various carbon fluxes in freshwater ecosystems.[4] High levels of freshwater acidification is harmful to various aquatic organisms. Nonetheless, there are many freshwater systems, including the Great Lakes, where pH levels could be decreasing, most likely due to CO2 accumulation in the atmosphere, however, increased monitoring is necessary to determine the full effects of acidification on pH levels.[5]
Freshwater vs. ocean acidification
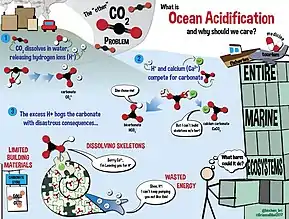
The ocean and the atmosphere are constantly exchanging massive amounts of CO2.[6] Over the last 800,000 years, the concentration of CO2 in the atmosphere has remained around 172-300 parts per million by volume (ppmv).[6] With increases of anthropogenic CO2 emissions, this number has risen to 387 ppmv in 2009.[6] From 2000-2008, 26% of anthropogenic CO2 was absorbed by the ocean.[6] CO2 is the primary factor affecting ocean pH, though other aspects can also play a role.[6] When dissolved in water, CO2 acts as a weak acid that primarily affects carbonate chemistry.[6] Dissolved CO2 increases the concentration of bicarbonate ions (HCO3−) and dissolved inorganic carbon (CT) as well as lowering pH levels.[6] Similar to oceans, freshwater bodies also absorb atmospheric CO2, lowering the pH of the water.[7] In addition to CO2, freshwater reservoir's pH values are altered by acid rain, nutrient runoff, and anthropogenic pollutants.[7] Freshwater takes up CO2 in the same mechanism as seawater; however, freshwater alkalinity is much more variable than seawater, due to differences in rocks present in the watershed and decreased salt concentrations.[7] Without this salt-buffer, pH changes in freshwater tend to be more pronounced than in ocean water. In freshwater systems, newly released H+ ions are not buffered by as many bicarbonate (HCO3−) ions as ocean water. Therefore, freshwater biota tends to have a higher evolutionary pH tolerance than seawater biota.[7]
Causes
CO2
Carbon dioxide reacts with water to form carbonic acid, bicarbonate, carbonate, and acidic protons via the following equilibria:
CO2 (aq) + H2O ⇌ H2CO3 ⇌ HCO3− + H+ ⇌ CO32− + 2 H+[8]
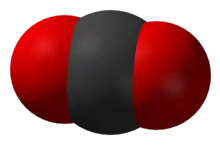
Dissociation of carbonic acid decreases the pH of the solution.[9] The degree of dissociation is controlled by the overall chemistry of the solution; in particular, alkalinity and temperature are primary controlling factors.[9] There has been a clear increase of pCO2 in some freshwater ecosystems in the last century due to anthropogenic influence that is contributing to freshwater acidification.[6] It is often difficult to quantify the role of increasing pCO2 (partial pressure) in freshwater due to the various sources of carbon dioxide and the many factors that affect it, such as the surrounding landscape, climate, organisms present, the water's chemistry, and biological processes (e.g. photosynthesis, respiration).[7] The dominant type of inorganic carbon present in freshwater indicates pH levels as more CO32- is present in basic water and free CO2 is in acidic water.[7] When the latter dissolves into the surface of freshwater it reacts to form carbonic acid.[7] Along with the overall trend of increasing CO2 in the atmosphere that is being absorbed by bodies of water, the levels of carbon dioxide fluctuate daily and seasonally.[10]
SOx and NOx
Two of the other main contributors to freshwater acidification are sulfur oxides and nitric oxides.[11] The accelerated burning of fossil fuels over the past two centuries has largely contributed to the acidification of freshwater ecosystems.[11] International cooperation and environmental legislation have reduced SOx and NOx in recent decades, as sulfate emissions peaked in the 1970s, with nitrogen following behind 10 years later.[11] High levels of sulfate concentration in runoff due to increased acidity inputs, coupled with both an increase in base cation run-off and a decrease of bicarbonate, creates the acidifying effects in aquatic systems.[12] Acidic rain seeps into and reacts with clay particles in the soil which leads to the leaching of aluminum into nearby bodies of water. Thus, as the pH levels decrease, aluminum levels will increase.[13] The higher levels of aluminum pose a risk of contamination to drinking water which can lead to several health diseases.[13] This creates a toxic environment for marine species and their habitat which can lead to extinction, reductions in population size, and an overall decrease in biodiversity.[13] Most of the nitrogen in its natural state found in terrestrial ecosystems will be utilized by vegetation. However, in large amounts, not all of it can be taken up by vegetation so the excess gets washed away with runoff in the form of nitrate, contributing to acidification in the same manner as sulfate.[12]
Buffering capacity
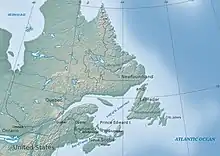
The buffering capacity of ecosystems helps them resist changes in pH. When this is lacking, it can lead to the acidification of freshwater reservoirs.[14] For example, the Atlantic region of Canada has the lowest acid deposition rates in Eastern North America, yet it has the most acidic waters on the continent.[14] This is due to the low buffering capacity of the regional bedrock and the addition of natural organic acids produced from close by wetlands.[14] Specifically, in Southwestern and Eastern Nova Scotia, there is a combination of high organic acidity, poor buffering, and high acid deposition to produce very low surface water pH levels and acid neutralization capacity (ANC) values.[14] In most of the Atlantic region, granite and shale bedrock are found, which contain very little buffering material.[14] Soil formed from low-buffering materials and the waters that drain from them are, therefore, susceptible to acidification, even under low acid deposition.[14] Soil that undergoes acidification can, in turn, have negative repercussions on agriculture.[15] Some species are able to withstand low pH levels in their environment. For example, frogs and perches can withstand a pH level of 4.[16] This allows these species to be unaffected by the acid deposition in their aquatic environment, allowing them to survive in these conditions.[16] However, most aquatic species, such as clams and snails, are unable to withstand low pH levels which negatively impacts their growth and survival. The high acidic levels deteriorate their thick shells decreasing their protection from predators.[16]
Harmful effects on aquatic ecosystems
%252C_Veenpluis_(Eriophorum_angustifolium)_03.JPG.webp)
Acidification of freshwater ecosystems may have significant negative effects. Changes in pH as a result of freshwater acidification imposes physiological challenges on individual organisms, may decrease native biodiversity, and can alter ecosystem structure and function entirely.[12] Macro-invertebrates and large vertebrates alike are particularly sensitive to acidification as they exhibit higher mortality and lower reproductive rates under acidified conditions.[12] These species are forced to expend more energy on buffering their body conditions to retain a livable pH and, therefore, must limit energy expenditure on processes such as hunting, sheltering, and reproducing.[12] Thus, embryonic development, and species' success, is also compromised in acidified freshwaters.[12]
Conversely, algae thrive in acidified environments, and may quickly dominate these habitats, outcompeting other species.[12] In most acidic freshwater reservoirs, there is an increase in the development of mosses and algae.[12] In particular, it is common to see an increase in the abundance of the sphagnum.[12] Sphagnum has a high capacity to exchange H+ for basic cations within freshwater. The thick layer of sphagnum restricts the exchange between surface water and sediment, further contributing to reduction in nutrient cycling in the ecosystem.[12]
Aquatic biomonitoring can be used to examine the health of aquatic ecosystems by assessing water quality and temperature.
Reducing acidification
Current and emerging chemical techniques
There are processes that can remediate the acidification of freshwaters. Liming is one such practice, where calcium carbonate (CaCO3) is added to these systems.[17] Liming aids freshwater chemical and biological recovery by increasing pH levels and essentially helping the habitat return to a similar condition to how it was prior to acidification.[17] Otherwise, recovery on its own would be very extensive and take a lot longer to achieve. When added to rivers, liming showed some positive effects on wildlife, increasing the abundance of fish and acid-sensitive invertebrates.[17] However, these effects are variable. In fact, other ecosystems showed a decrease in invertebrate abundance.[17] New technologies have been developed to reduce emissions of nitrogen oxide and sulfur dioxide, linked to acid rain and water acidification. These include, wet lime, gypsum denitrification, Ammonia reduction denitrification, Electron beam irradiation desulfurization and denitrification, and Pulse plasma chemical desulfurization and denitrification.[15]
Government regulations and policies
A large decrease of acid rain and acidic bodies of water in the past couple of decades has been a direct result of governmental regulations on anthropogenic emissions, specifically SOx and NOx.[18] For example, the Canada-United States Air Quality Agreement has greatly reduced acid rain and ozone levels by 78% in Canada and 92% in the United States, as of 2020.[19] Moreover, investing in scientists to monitor and collect data is essential to create a model used to establish successful policies.[20] For instance, a protocol can be implemented to mitigate the issue.[20] Also, governments could invest funds to subsidize companies to decrease their pollution and incentivize them to use innovative methods of production, to lower both greenhouse gas emissions and the amount of acidic substances created. Furthermore, government institutions across the globe can connect on the issue of acidification and work together to find a feasible solution through international agreements.[15] Some successful government implementations include the Acid Rain Program[21] established in the United States in 1995, and the most recent Gothenburg Protocol, established by the United Nations Economic Commission for Europe (UNECE) to reduce acidification.[22]
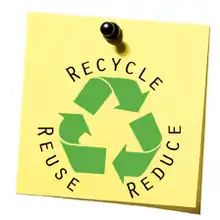
Public education
Another important factor to consider when looking at reducing freshwater acidification are the choices people make to protect the environment everyday. Having a basic understanding of environmental problems, such as climate change and acid rain, can influence people to act differently by being more conscious of these issues. Following a circular approach to reduce, reuse and recycle can reduce resource depletion and waste minimization, including decreasing water acidity.[23] Also, establishing school programs to ensure children learn from a young age the importance of sustainability and environmental protection. Moreover, the practice of waste separation is fundamental as it allows for the breakdown of the chemicals that cause acid rain.[15] And, finally, being altogether more aware of the effect human actions have on the environment to better protect the planet.[15]
References
- Psenner, Roland (March 1994). "Environmental impacts on freshwaters: acidification as a global problem". Science of the Total Environment. 143 (1): 53–61. Bibcode:1994ScTEn.143...53P. doi:10.1016/0048-9697(94)90532-0. ISSN 0048-9697.
- Irwin, J.G.; Williams, M.L. (1988). "Acid rain: Chemistry and transport". Environmental Pollution. 50 (1–2): 29–59. doi:10.1016/0269-7491(88)90184-4. ISSN 0269-7491. PMID 15092652.
- Jean-Pierre Gattuso; Lina Hansson, eds. (2011). Ocean acidification. Oxford University Press. ISBN 9780199591084. OCLC 975179973.
- "Measurements and observations : OCB-OA". Whoi.edu. Retrieved 2019-03-24.
- Phillips, Jennifer C.; McKinley, Galen A.; Bennington, Val; Bootsma, Harvey A.; Pilcher, Darren J.; Sterner, Robert W.; Urban, Noel R. (2015). "The Potential for CO₂-Induced Acidification in Freshwater: A Great Lakes Case Study". Oceanography. 28 (2): 136–145. doi:10.5670/oceanog.2015.37. ISSN 1042-8275. JSTOR 24861876.
- Weiss, Linda C.; Pötter, Leonie; Steiger, Annika; Kruppert, Sebastian; Frost, Uwe; Tollrian, Ralph (January 2018). "Rising pCO2 in Freshwater Ecosystems Has the Potential to Negatively Affect Predator-Induced Defenses in Daphnia". Current Biology. 28 (2): 327–332.e3. doi:10.1016/j.cub.2017.12.022. ISSN 0960-9822. PMID 29337079.
- Hasler, Caleb T.; Butman, David; Jeffrey, Jennifer D.; Suski, Cory D. (January 2016). Sterner, Robert (ed.). "Freshwater biota and rising pCO 2 ?". Ecology Letters. 19 (1): 98–108. doi:10.1111/ele.12549. PMID 26610406.
- U.S. Department of Commerce National Oceanic & Atmospheric Administration. "Lesson 3: Ocean Acidification" (PDF).
{{cite journal}}
: Cite journal requires|journal=
(help) - Mitchell, Mark J.; Jensen, Oliver E.; Cliffe, K. Andrew; Maroto-Valer, M. Mercedes (2009). ""A model of carbon dioxide dissolution and mineral carbonation kinetics"". Proceedings of the Royal Society A. 466 (2117): 1265–1290. doi:10.1098/rspa.2009.0349. S2CID 33658865.
- Muniz, Ivar P. (1990). "Freshwater acidification: its effects on species and communities of freshwater microbes, plants and animals". Proceedings of the Royal Society of Edinburgh, Section B: Biological Sciences. 97: 227–254. doi:10.1017/s0269727000005364. ISSN 0269-7270.
- Cardoso, A.C.; Free, G.; Nõges, P.; Kaste, Ø.; Poikane, S.; Solheim, A. Lyche (2009). "Lake Management, Criteria". Encyclopedia of Inland Waters. Elsevier. pp. 310–331. doi:10.1016/b978-012370626-3.00244-1. ISBN 9780123706263.
- Henriksen, Arne; Kämäri, Juha; Posch, Maximilian; Wilander, Anders (1992). "Critical Loads of Acidity: Nordic Surface Waters". Ambio. 21 (5): 356–363. ISSN 0044-7447. JSTOR 4313961.
- "Acid Rain, Aluminum Link Found". Washington Post. Retrieved 19 April 2022.
- Clair, Thomas A.; Dennis, Ian F.; Scruton, David A.; Gilliss, Mallory (December 2007). "Freshwater acidification research in Atlantic Canada: a review of results and predictions for the future". Environmental Reviews. 15 (NA): 153–167. doi:10.1139/a07-004. ISSN 1181-8700.
- Chen, Changan; Lin, Juntong; Liu, Yuhang; Ren, Xiangru (2022). "Effects of freshwater acidification and countermeasures". IOP Conference Series: Earth and Environmental Science. 1011: 012035. doi:10.1088/1755-1315/1011/1/012035. S2CID 248122033.
- "Effects of Acid Rain - Surface Waters and Aquatic Animals" (PDF). Landuse.alberta.ca. Retrieved 19 April 2022.
- Mant, Rebecca C.; Jones, David L.; Reynolds, Brian; Ormerod, Steve J.; Pullin, Andrew S. (2013-08-01). "A systematic review of the effectiveness of liming to mitigate impacts of river acidification on fish and macro-invertebrates". Environmental Pollution. 179: 285–293. doi:10.1016/j.envpol.2013.04.019. ISSN 0269-7491. PMID 23707951.
- Menz, Fredric C.; Seip, Hans M. (2004-08-01). "Acid rain in Europe and the United States: an update". Environmental Science & Policy. 7 (4): 253–265. doi:10.1016/j.envsci.2004.05.005. ISSN 1462-9011.
- Canada, Environment and Climate Change (2005-01-25). "Canada-United States Air Quality Agreement: overview". www.canada.ca. Retrieved 2023-03-25.
- Grennfelt, Peringe; Engleryd, Anna; Forsius, Martin; Hov, Øystein; Rodhe, Henning; Cowling, Ellis (2020-04-01). "Acid rain and air pollution: 50 years of progress in environmental science and policy". Ambio. 49 (4): 849–864. doi:10.1007/s13280-019-01244-4. ISSN 1654-7209. PMC 7028813. PMID 31542884.
- US EPA, OAR (2014-08-21). "Acid Rain Program". www.epa.gov. Retrieved 2023-03-24.
- "Protocol to Abate Acidification, Eutrophication and Ground-level Ozone | UNECE". unece.org. Retrieved 2023-03-25.
- Morseletto, Piero (2020-02-01). "Targets for a circular economy". Resources, Conservation and Recycling. 153: 104553. doi:10.1016/j.resconrec.2019.104553. hdl:1871.1/c4c1f149-0a90-46fb-9105-cbf7157cf3f8. ISSN 0921-3449. S2CID 209343855.