Industrial symbiosis
Industrial symbiosis[1] a subset of industrial ecology. It describes how a network of diverse organizations can foster eco-innovation and long-term culture change, create and share mutually profitable transactions—and improve business and technical processes.
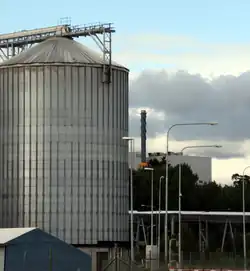
Although geographic proximity is often associated with industrial symbiosis, it is neither necessary nor sufficient—nor is a singular focus on physical resource exchange. Strategic planning is required to optimize the synergies of co-location. In practice, using industrial symbiosis as an approach to commercial operations—using, recovering and redirecting resources for reuse—results in resources remaining in productive use in the economy for longer. This in turn creates business opportunities, reduces demands on the earth's resources, and provides a stepping-stone towards creating a circular economy.[2]
Industrial symbiosis is a subset of industrial ecology, with a particular focus on material and energy exchange. Industrial ecology is a relatively new field that is based on a natural paradigm, claiming that an industrial ecosystem may behave in a similar way to the natural ecosystem wherein everything gets recycled, albeit the simplicity and applicability of this paradigm has been questioned.[3]
Introduction
Eco-industrial development is one of the ways in which industrial ecology contributes to the integration of economic growth and environmental protection. Some of the examples of eco-industrial development are:
- Circular economy (single material and/or energy exchange)
- Greenfield eco-industrial development (geographically confined space)
- Brownfield eco-industrial development (geographically confined space)
- Eco-industrial network (no strict requirement of geographical proximity)
- Virtual eco-industrial network (networks spread in large areas e.g. regional network)
- Networked Eco-industrial System (macro level developments with links across regions)
Industrial symbiosis engages traditionally separate industries in a collective approach to competitive advantage involving physical exchange of materials, energy,[4] water,[5] and/or by-products.[6] The keys to industrial symbiosis are collaboration and the synergistic possibilities offered by geographic proximity".[7] Notably, this definition and the stated key aspects of industrial symbiosis, i.e., the role of collaboration and geographic proximity, in its variety of forms, has been explored and empirically tested in the UK through the research and published activities of the National Industrial Symbiosis Programme.[8][9][10]
Industrial symbiosis systems collectively optimize material and energy use at efficiencies beyond those achievable by any individual process alone. IS systems such as the web of materials and energy exchanges among companies in Kalundborg, Denmark have spontaneously evolved from a series of micro innovations over a long time scale;[11] however, the engineered design and implementation of such systems from a macro planner's perspective, on a relatively short time scale, proves challenging.
Often, access to information on available by-products is difficult to obtain.[12] These by-products are considered waste and typically not traded or listed on any type of exchange. Only a small group of specialized waste marketplaces addresses this particular kind of waste trading.[13]
Example
Recent work reviewed government policies necessary to construct a multi-gigaWatt photovoltaic factory and complementary policies to protect existing solar companies are outlined and the technical requirements for a symbiotic industrial system are explored to increase the manufacturing efficiency while improving the environmental impact of solar photovoltaic cells. The results of the analysis show that an eight-factory industrial symbiotic system can be viewed as a medium-term investment by any government, which will not only obtain direct financial return, but also an improved global environment.[14] This is because synergies have been identified for co-locating glass manufacturing and photovoltaic manufacturing.[15]
The waste heat from glass manufacturing can be used in industrial-sized greenhouses for food production.[16] Even within the PV plant itself a secondary chemical recycling plant can reduce environmental impact while improving economic performance for the group of manufacturing facilities.[17]
In DCM Shriram consolidated limited (Kota unit) produces caustic soda, calcium carbide, cement and PVC resins. Chlorine and hydrogen are obtained as by-products from caustic soda production, while calcium carbide produced is partly sold and partly is treated with water to form slurry(aqueous solution of calcium hydroxide) and ethylene. The chlorine and ethylene produced are utilised to form PVC compounds, while the slurry is consumed for cement production by wet process. Hydrochloric acid is prepared by direct synthesis where the pure chlorine gas can be combined with hydrogen to produce hydrogen chloride in the presence of UV light.[18]
References
- Lombardi, D. Rachel; Laybourn, Peter (February 2012). "Redefining Industrial Symbiosis". Journal of Industrial Ecology. 16 (1): 28–37. doi:10.1111/j.1530-9290.2011.00444.x. S2CID 55804558.
- Fraccascia, Luca; Giannoccaro, Ilaria (June 2020). "What, where, and how measuring industrial symbiosis: A reasoned taxonomy of relevant indicators". Resources, Conservation and Recycling. 157: 104799. doi:10.1016/j.resconrec.2020.104799.
- Jensen, Paul D.; Basson, Lauren; Leach, Matthew (October 2011). "Reinterpreting Industrial Ecology" (PDF). Journal of Industrial Ecology. 15 (5): 680–692. doi:10.1111/j.1530-9290.2011.00377.x. S2CID 9188772.
- Fraccascia, Luca; Yazdanpanah, Vahid; van Capelleveen, Guido; Yazan, Devrim Murat (30 June 2020). "Energy-based industrial symbiosis: a literature review for circular energy transition". Environment, Development and Sustainability. 23 (4): 4791–4825. doi:10.1007/s10668-020-00840-9. ISSN 1573-2975.
- Tiu, Bryan Timothy C.; Cruz, Dennis E. (1 April 2017). "An MILP model for optimizing water exchanges in eco-industrial parks considering water quality". Resources, Conservation and Recycling. Sustainable development paths for resource-constrained process industries. 119: 89–96. doi:10.1016/j.resconrec.2016.06.005. ISSN 0921-3449.
- Jacobsen, Noel Brings (2006). "Industrial Symbiosis in Kalundborg, Denmark: A Quantitative Assessment of Economic and Environmental Aspects". Journal of Industrial Ecology. 10 (1–2): 239–255. doi:10.1162/108819806775545411. ISSN 1530-9290. S2CID 153973389.
- Chertow, Marian R. (November 2000). "Industrial Symbiosis: Literature and Taxonomy". Annual Review of Energy and the Environment. 25 (1): 313–337. doi:10.1146/annurev.energy.25.1.313.
- Jensen, Paul D.; Basson, Lauren; Hellawell, Emma E.; Bailey, Malcolm R.; Leach, Matthew (May 2011). "Quantifying 'geographic proximity': Experiences from the United Kingdom's National Industrial Symbiosis Programme" (PDF). Resources, Conservation and Recycling. 55 (7): 703–712. doi:10.1016/j.resconrec.2011.02.003.
- Lombardi, D. Rachel; Laybourn, Peter (February 2012). "Redefining Industrial Symbiosis". Journal of Industrial Ecology. 16 (1): 28–37. doi:10.1111/j.1530-9290.2011.00444.x. S2CID 55804558.
- Jensen, Paul D. (February 2016). "The role of geospatial industrial diversity in the facilitation of regional industrial symbiosis" (PDF). Resources, Conservation and Recycling. 107: 92–103. doi:10.1016/j.resconrec.2015.11.018.
- Ehrenfeld, John; Gertler, Nicholas (December 1997). "Industrial Ecology in Practice: The Evolution of Interdependence at Kalundborg". Journal of Industrial Ecology. 1 (1): 67–79. doi:10.1162/jiec.1997.1.1.67. S2CID 8076213.
- Fraccascia, Luca; Yazan, Devrim Murat (September 2018). "The role of online information-sharing platforms on the performance of industrial symbiosis networks". Resources, Conservation and Recycling. 136: 473–485. doi:10.1016/j.resconrec.2018.03.009.
- van Capelleveen, Guido; Amrit, Chintan; Yazan, Devrim Murat (2018). Otjacques, Benoît; Hitzelberger, Patrik; Naumann, Stefan; Wohlgemuth, Volker (eds.). "A Literature Survey of Information Systems Facilitating the Identification of Industrial Symbiosis". From Science to Society. Progress in IS. Cham: Springer International Publishing: 155–169. doi:10.1007/978-3-319-65687-8_14. ISBN 978-3-319-65687-8.
- Pearce, Joshua M. (May 2008). "Industrial symbiosis of very large-scale photovoltaic manufacturing" (PDF). Renewable Energy. 33 (5): 1101–1108. doi:10.1016/j.renene.2007.07.002. S2CID 18310744.
- Nosrat, Amir H.; Jeswiet, Jack; Pearce, Joshua M. (2009). "Cleaner production via industrial symbiosis in glass and largescale solar photovoltaic manufacturing". 2009 IEEE Toronto International Conference Science and Technology for Humanity (TIC-STH). pp. 967–970. doi:10.1109/TIC-STH.2009.5444358. ISBN 978-1-4244-3877-8. S2CID 34736473.
- Andrews, R.; Pearce, J.M. (September 2011). "Environmental and economic assessment of a greenhouse waste heat exchange" (PDF). Journal of Cleaner Production. 19 (13): 1446–1454. doi:10.1016/j.jclepro.2011.04.016. S2CID 53997847.
- Kreiger, M.A.; Shonnard, D.R.; Pearce, J.M. (January 2013). "Life cycle analysis of silane recycling in amorphous silicon-based solar photovoltaic manufacturing". Resources, Conservation and Recycling. 70: 44–49. doi:10.1016/j.resconrec.2012.10.002. S2CID 3961031.
- DSCL Annual Report , 2011-12 (PDF). pp. 22–23. Archived from the original (PDF) on 1 August 2014. Retrieved 18 May 2015.