Madin-Darby canine kidney cells
Madin-Darby canine kidney (MDCK) cells are a model mammalian cell line used in biomedical research. MDCK cells are used for a wide variety of cell biology studies including cell polarity, cell-cell adhesions (termed adherens junctions), collective cell motility, toxicity studies,[1] as well as responses to growth factors. It is one of few cell culture models that is suited for 3D cell culture and multicellular rearrangements known as branching morphogenesis.[2]
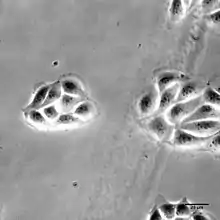
History
Following the initial isolation in 1958 of epithelial cells from the kidney tubule of an adult Cocker Spaniel dog by Stewart H. Madin and Norman B. Darby, Jr.,[3] the cell line bearing their name was employed primarily as a model for viral infection of mammalian cells.[4][5][6] Indeed, they chose to isolate kidney tubules with precisely this goal in mind, as they had previously succeeded with viral infection of cells derived from kidney tubules from other mammals.[7] Thus the initial goal in isolating and culturing cells from this tissue was not to generate a new model system for epithelial cell biology. It was not until 1970 that the laboratory of Zbynek Brada published work describing MDCK cells as a representative cell line bearing hallmarks of kidney tubule epithelial cells.[8] They based this conclusion on the fluid transport activities of monolayers formed of MDCK cells, the presence of microvilli on their apical (upper) surface, and their ability to self-organize, when grown in 3D, into hollow spheres. In their report, the authors speculated that the "histotypic expression" by which MDCK cells formed structures reminiscent of their tissue of origin might be fruitfully applied to the study of other tissues. The following decades have proved them largely right, although the repertoire for studying the organization and behavior of cells within tissues has vastly expanded.[9]
Through the 1970s, the MDCK cell line found new use as a model for mammalian epithelial tissue. In 1982 Mina Bissell and colleagues showed that MDCK monolayers responded to the addition of a collagen overlay (dubbed a "sandwich culture") by proliferating and forming hollow tubules.[10] This hinted for the first time that the cell line would respond to 3D environments by self-organizing into the appropriate 3D structure reminiscent of kidney tubules. In the following years, the culture of MDCK cells embedded fully in collagen was shown to yield hollow spheres, or acini.[11] These were simple epithelial monolayers with a defined interior and exterior. However, the fact that MDCK cells did not form tubules under these conditions remained unexplained until later.
Over the same period in the 1980s, biologists studying cell motility had hit upon an interesting and reproducible behavior of cells in culture: the scattering response. Epithelial cells in culture grow normally as tight clusters. However, they could be induced to break cell-cell contacts and become elongated and motile after exposure to a "scatter factor" that was secreted by mesenchymal cells such as Swiss 3T3 fibroblasts.[12] This was best described by Julia Gray's group in 1987.[13] During the same period in the mid 1980s, a monoclonal antibody was reported by the group of Walter Birchmeier to disrupt cell-cell contacts and alter the front-rear polarity of cells in culture.[14][15] The target of this antibody was later identified as a component of cell-cell junctions, E-cadherin.[16] These disparate observations eventually coalesced into a resilient paradigm for cell motility and cell polarity. Epithelial cells are typically nonmotile, but can become motile by inhibiting cell-cell junctions or by addition of growth factors that induce scattering.[17] Both of these are reversible, and both involve the rupture of cell-cell junctions.
In 1991, the response of MDCK acini in 3D culture to the scatter factor was first reported by Lelio Orci and colleagues.[18] They cultured acini of MDCK cells in collagen gels with or without Swiss 3T3 fibroblasts, in which media could exchange but the cell types were not in direct contact. This cell culture strategy, termed coculture, induced MDCK acini to undergo branching morphogenesis, in which cells rearrange into a network of interconnected tubules that resembles the development of many tissues.[19] In the same year, the "scatter factor" was shown to be a previously described protein secreted by fibroblasts, hepatocyte growth factor (HGF).[20] This work solved an outstanding mystery of MDCK culture, as the tissue from which these cells were derived is tubular, yet they had previously only developed into spherical acini in 3D culture. Beyond that immediate paradox, a crucial connection was forged between the acute induction of cell motility in 2D culture by the "scatter factor", and its impact on the spatial organization adopted by tissues in 3D. This connection remains significant as a link between precisely defined mechanisms of cell motility in 2D and complex rearrangements in 3D whose regulation is yet to be understood fully.
Branching morphogenesis
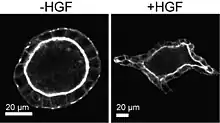
In the last 20 years, understanding of MDCK cell biology in 3D culture has been advanced most notably by the laboratory of Keith Mostov. This group has focused on the regulation of cell polarity and its downstream effects on branching morphogenesis.[21][22] Indeed, the body of work generated by the Mostov group has successfully synthesized decades of knowledge about the spatial segregation of cellular functions, and their molecular markers, into a remarkable model for the generation and homeostasis of cellular polarity in tissues.[23][24] In 2003 the Mostov group reported the first comprehensive account linking branching morphogenesis with hallmarks of apical-basal polarity.[25] This work established that MDCK cells do not lose contacts with neighbors during the onset of branching morphogenesis, but that canonical markers of cell polarity are transiently lost. One outcome of this shift in polarity is the reorientation of cell division along a newly growing branch of cells, in order to correctly position daughter cells to continue branch extension. Cell motility by which MDCK cells produce and elongate branches was linked with these polarity changes.
These findings were integrated into a model for branching morphogenesis focused on the transient rearrangement of cell polarity signaling. This model has informally been referred to as the Mostov pathway. This allows normally nonmotile cells to generate protrusions and migrate collectively, followed by redifferentiation and formation of hollow tubules. In support of this model, Mostov and colleagues have identified the effects of HGF on MDCK acini as eliciting a partial transition from epithelial to mesenchymal cell phenotypes.[26] This argument marshals an established signaling program termed the epithelial to mesenchymal transition (EMT), by which sessile epithelial cells become motile and break cell-cell contacts.[17] EMT has been proposed as the transcriptional signaling cascade that drives cell scattering, although previously researchers did not conflate the two.[27][28] Given the distinction that, for acini in 3D, cell-cell junctions do not rupture, it is unclear how to precisely relate the EMT concept with branching morphogenesis.
The Mostov group has also investigated the means by which HGF activates cell motility during MDCK branching morphogenesis.[29][30] Their studies have shown that branching morphogenesis requires the Erk transcription factor, downstream of the mitogen activated protein kinase cascade, a well-defined signal transduction pathway implicated in cell motility and proliferation.[31] The precise cell motility machinery responsible for MDCK branching morphogenesis has not been specified by the Mostov group, beyond the requirement for a signaling protein involved in regulating the small GTPase Rho.[30] Moreover, the Gardel lab has shown that invasive motility of MDCK cells in acini requires Dia1, which regulates cell adhesions to individual collagen fibrils.[32]
Meanwhile, other groups have demonstrated the requirement for cell-ECM adhesion proteins or their regulators in MDCK branching morphogenesis.[33][34] Using a modified protocol for MDCK cell culture and branching morphogenesis, Gierke and Wittman established the requirement for microtubule dynamics in regulating the early steps in branching.[35] They observed deficient cell adhesive coupling to the collagen matrix when microtubules were deregulated. This phenotype indicated the importance of trafficking the appropriate cell adhesion and protrusion proteins to the cell front as branching morphogenesis was initiated. Combined with observations from the Mostov group, this work confirmed that cell polarity is indispensable for MDCK acinar homeostasis as well as migratory behaviors during branching morphogenesis.
References
- Lindsay CD (November 1996). "Assessment of aspects of the toxicity of Clostridium perfringens epsilon-toxin using the MDCK cell line". Hum Exp Toxicol. 15 (11): 904–908. doi:10.1177/096032719601501107. PMID 8938486. S2CID 21968438.
- Erin O'Brien, Lucy; Zegers, Mirjam M. P.; Mostov, Keith E. (2002). "Building epithelial architecture: insights from three-dimensional culture models". Nature Reviews Molecular Cell Biology. 3 (7): 531–537. doi:10.1038/nrm859. PMID 12094219. S2CID 13410353.
- "ATCC". ATCC. Retrieved 28 August 2017.
- Green, I. J. (1962-10-05). "Serial Propagation of Influenza B (Lee) Virus in a Transmissible Line of Canine Kidney Cells". Science. 138 (3536): 42–43. Bibcode:1962Sci...138...42G. doi:10.1126/science.138.3536.42. ISSN 0036-8075. PMID 13901412. S2CID 30459359.
- Gaush, Charles R.; Hard, Walter L.; Smith, Thomas F. (1966-07-01). "Characterization of an Established Line of Canine Kidney Cells (MDCK)". Proceedings of the Society for Experimental Biology and Medicine. 122 (3): 931–935. doi:10.3181/00379727-122-31293. ISSN 0037-9727. PMID 5918973. S2CID 44521872.
- Moulton, J.E.; Frazier, L.M. (1961). "Deoxyribonucleic acid and protein changes in dog kidney cells infected with infectious canine hepatitis virus". Virology. 15 (2): 91–101. doi:10.1016/0042-6822(61)90226-4. PMID 14476648.
- Karl Matlin, PhD, personal communication
- Leighton, J; Estes, LW; Mansukhani, S; Brada, Z (1970). "A cell line derived from normal dog kidney (MDCK) exhibiting qualities of papillary adenocarcinoma and of renal tubular epithelium". Cancer. 26 (5): 1022–8. doi:10.1002/1097-0142(197011)26:5<1022::aid-cncr2820260509>3.0.co;2-m. PMID 4248968.
- Shamir, ER; Ewald, AJ (2014). "Three-dimensional organotypic culture: experimental models of mammalian biology and disease". Nat Rev Mol Cell Biol. 15 (10): 647–64. doi:10.1038/nrm3873. PMC 4352326. PMID 25237826.
- Hall, HG; Farson, DA; Bissell, MJ (1982). "Lumen formation by epithelial cell lines in response to collagen overlay: a morphogenetic model in culture". Proc Natl Acad Sci U S A. 79 (15): 4672–6. Bibcode:1982PNAS...79.4672H. doi:10.1073/pnas.79.15.4672. PMC 346738. PMID 6956885.
- McAteer, James A.; Evan, Andrew P.; Gardner, Kenneth D. (1987). "Morphogenetic clonal growth of kidney epithelial cell line MDCK". The Anatomical Record. 217 (3): 229–239. doi:10.1002/ar.1092170303. PMID 3578840. S2CID 6379141.
- Stoker, M; Perryman, M (1985). "An epithelial scatter factor released by embryo fibroblasts". J Cell Sci. 77: 209–23. doi:10.1242/jcs.77.1.209. PMID 3841349.
- Stoker, Michael; Gherardi, Ermanno; Perryman, Marion; Gray, Julia (1987). "Scatter factor is a fibroblast-derived modulator of epithelial cell mobility". Nature. 327 (6119): 239–242. Bibcode:1987Natur.327..239S. doi:10.1038/327239a0. PMID 2952888. S2CID 39458594.
- Behrens, J; Birchmeier, W; Goodman, SL; Imhof, BA (1985). "Dissociation of Madin-Darby canine kidney epithelial cells by the monoclonal antibody anti-arc-1: mechanistic aspects and identification of the antigen as a component related to uvomorulin". J Cell Biol. 101 (4): 1307–15. doi:10.1083/jcb.101.4.1307. PMC 2113935. PMID 2995405.
- Imhof, Beat A.; Vollmers, H. Peter; Goodman, Simon L.; Birchmeier, Walter (1983). "Cell-cell interaction and polarity of epithelial cells: specific perturbation using a monoclonal antibody". Cell. 35 (3): 667–675. doi:10.1016/0092-8674(83)90099-5. PMID 6652682. S2CID 41850671.
- Behrens, J; Mareel, MM; Van Roy, FM; Birchmeier, W (1989). "Dissecting tumor cell invasion: epithelial cells acquire invasive properties after the loss of uvomorulin-mediated cell-cell adhesion". J Cell Biol. 108 (6): 2435–47. doi:10.1083/jcb.108.6.2435. PMC 2115620. PMID 2661563.
- Paul Thiery, Jean (2009). "Epithelial-mesenchymal transitions in development and disease". Cell. 139 (5): 871–890. doi:10.1016/j.cell.2009.11.007. PMID 19945376.
- Montesano, R.; Schaller, G.; Orci, L. (1991). "Induction of epithelial tubular morphogenesis in vitro by fibroblast-derived soluble factors". Cell. 66 (4): 697–711. doi:10.1016/0092-8674(91)90115-F. PMID 1878968. S2CID 6309985.
- Affolter, Markus; Zeller, Rolf; Caussinus, Emmanuel (2009). "Tissue remodelling through branching morphogenesis". Nature Reviews Molecular Cell Biology. 10 (12): 831–842. doi:10.1038/nrm2797. PMID 19888266. S2CID 5960255.
- Weidner, KM; Arakaki, N; Hartmann, G; Vandekerckhove, J; Weingart, S; Rieder, H; Fonatsch, C; Tsubouchi, H; Hishida, T; Daikuhara, Y (1991). "Evidence for the identity of human scatter factor and human hepatocyte growth factor". Proc Natl Acad Sci U S A. 88 (16): 7001–5. Bibcode:1991PNAS...88.7001W. doi:10.1073/pnas.88.16.7001. PMC 52221. PMID 1831266.
- Bryant, DM; Mostov, KE (2008). "From cells to organs: building polarized tissue". Nat Rev Mol Cell Biol. 9 (11): 887–901. doi:10.1038/nrm2523. PMC 2921794. PMID 18946477.
- Erin O'Brien, Lucy; Zegers, Mirjam M. P.; Mostov, Keith E. (2002). "Building epithelial architecture: insights from three-dimensional culture models". Nature Reviews Molecular Cell Biology. 3 (7): 531–537. doi:10.1038/nrm859. PMID 12094219. S2CID 13410353.
- Martin-Belmonte, Fernando; Gassama, Ama; Datta, Anirban; Yu, Wei; Rescher, Ursula; Gerke, Volker; Mostov, Keith (2007). "PTEN-mediated apical segregation of phosphoinositides controls epithelial morphogenesis through Cdc42". Cell. 128 (2): 383–397. doi:10.1016/j.cell.2006.11.051. PMC 1865103. PMID 17254974.
- Bryant, David M.; Roignot, Julie; Datta, Anirban; Overeem, Arend W.; Kim, Minji; Yu, Wei; Peng, Xiao; Eastburn, Dennis J.; Ewald, Andrew J.; Werb, Zena; Mostov, Keith E. (2014). "A molecular switch for the orientation of epithelial cell polarization". Developmental Cell. 31 (2): 171–187. doi:10.1016/j.devcel.2014.08.027. PMC 4248238. PMID 25307480.
- Yu, Wei; O'Brien, Lucy E.; Wang, Fei; Bourne, Henry; Mostov, Keith E.; Zegers, Mirjam M.P. (2003). "Hepatocyte growth factor switches orientation of polarity and mode of movement during morphogenesis of multicellular epithelial structures". Molecular Biology of the Cell. 14 (2): 748–763. doi:10.1091/mbc.E02-06-0350. PMC 150005. PMID 12589067.
- Zegers, Mirjam M.P.; O'Brien, Lucy E.; Yu, Wei; Datta, Anirban; Mostov, Keith E. (2003). "Epithelial polarity and tubulogenesis in vitro". Trends in Cell Biology. 13 (4): 169–176. doi:10.1016/S0962-8924(03)00036-9. PMID 12667754.
- Janda, Elzbieta; Lehmann, Kerstin; Killisch, Iris; Jechlinger, Martin; Herzig, Michaela; Downward, Julian; Beug, Hartmut; Grünert, Stefan (2002). "Ras and TGFβ cooperatively regulate epithelial cell plasticity and metastasis". The Journal of Cell Biology. 156 (2): 299–314. doi:10.1083/jcb.200109037. PMC 2199233. PMID 11790801.
- Kalluri, Raghu (2003). "Epithelial-mesenchymal transition and its implications for fibrosis". Journal of Clinical Investigation. 112 (12): 1776–1784. doi:10.1172/JCI20530. PMC 297008. PMID 14679171.
- Erin O'Brien, Lucy (2004). "ERK and MMPs sequentially regulate distinct stages of epithelial tubule development". Developmental Cell. 7 (1): 21–32. doi:10.1016/j.devcel.2004.06.001. PMID 15239951.
- Kim, M.; Shewan, A. M.; Ewald, A. J.; Werb, Z.; Mostov, K. E. (2015). "p114RhoGEF governs cell motility and lumen formation during tubulogenesis through a ROCK–myosin-II pathway". Journal of Cell Science. 128 (23): 4317–4327. doi:10.1242/jcs.172361. PMC 4712812. PMID 26483385.
- Vial, Emmanuel; Sahai, Erik; Marshall, Christopher J. (2003). "ERK-MAPK signaling coordinately regulates activity of Rac1 and RhoA for tumor cell motility". Cancer Cell. 4 (1): 67–79. doi:10.1016/S1535-6108(03)00162-4. PMID 12892714.
- Timothy Best (2017-05-06), Tim Fessenden Thesis Defense 05-02-2017, retrieved 2017-09-28
- Hunter, Michael P.; Zegers, Mirjam M. (2010). "Pak1 regulates branching morphogenesis in 3D MDCK cell culture by a PIX and β1-integrin-dependent mechanism". American Journal of Physiology. Cell Physiology. 299 (1): C21–C32. doi:10.1152/ajpcell.00543.2009. PMC 2904258. PMID 20457839.
- Jiang, Si-Tse; Chiu, Sue-Jean; Chen, Hong-Chen; Chuang, Woei-Jer; Tang, Ming-Jer (2001). "Role of α 3 β 1 integrin in tubulogenesis of Madin-Darby canine kidney cells". Kidney International. 59 (5): 1770–1778. doi:10.1046/j.1523-1755.2001.0590051770.x. PMID 11318947.
- Gierke, Sarah; Wittmann, Torsten (2012). "EB1-recruited microtubule+ TIP complexes coordinate protrusion dynamics during 3D epithelial remodeling". Current Biology. 22 (9): 753–762. doi:10.1016/j.cub.2012.02.069. PMC 3350573. PMID 22483942.