Ototoxic medication
Ototoxicity is defined as the toxic effect on the functioning of the inner ear, which may lead to temporary or permanent hearing loss (cochleotoxic) and balancing problems (vestibulotoxic).[1] Drugs or pharmaceutical agents inducing ototoxicity are regarded as ototoxic medications.
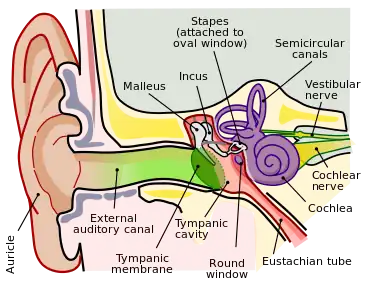
There is a wide range of ototoxic medications, for example, antibiotics, antimalarials, chemotherapeutic agents, non-steroidal anti-inflammatory drugs (NSAIDs) and loop diuretics.[2] While these drugs target on different body systems, they also trigger ototoxicity through different mechanisms, for example, destruction to cellular tissues of inner ear parts and disturbance on auditory nervous system.[2]
Onset of ototoxicity ranges from taking a single dose to long-term usage of the drugs.[3] Signs and symptoms of ototoxicity include tinnitus, hearing loss, dizziness and nausea and/or vomiting.[3] The diagnosis of medicine-induced ototoxicity is challenging as it usually shows only mild symptoms in early stages. Thus, prospective ototoxicity monitoring would be required when patients are using ototoxic medications.[1] Fortunately, the majority of ototoxicity cases are reversible by stopping the medication concerned.
Antibiotics and chemotherapeutic agents
The most common classes of ototoxic medications include antibiotics (including aminoglycosides and glycopeptides) and chemotherapeutic agents. Aminoglycosides and some chemotherapeutic agents are associated with both cochleotoxicity and vestibulotoxicity. They are thought to damage the hair cells of the cochlea. Long-term exposure to these drugs may cause damage that progresses to the upper turn of the cochlea, impairing hearing or even causing deafness.[4] Glycopeptides, on the other hand, are rarely associated with ototoxicity.

Aminoglycosides
Aminoglycosides are a class of antibiotics. The most frequently used aminoglycosides include gentamicin, amikacin and streptomycin. These antibiotics are usually used in combination with other antimicrobial agents to treat drug-resistant organisms. For example, they are used with β-lactam for bacterial infections in pneumonia.[5] They are usually given either intravenously or intramuscularly due to their poor oral absorption.
Aminoglycosides irreversibly inhibit protein synthesis of bacteria, which specifically helps kill the gram-negative bacteria. The drug is first transported into the bacterial cell and it binds to the 30S ribosomal subunit.[6] This action interferes with the reading of codons during mRNA translation, causing misreading and premature termination of the process. This inhibits protein synthesis and ultimately leads to the death of bacterial cells.[7]
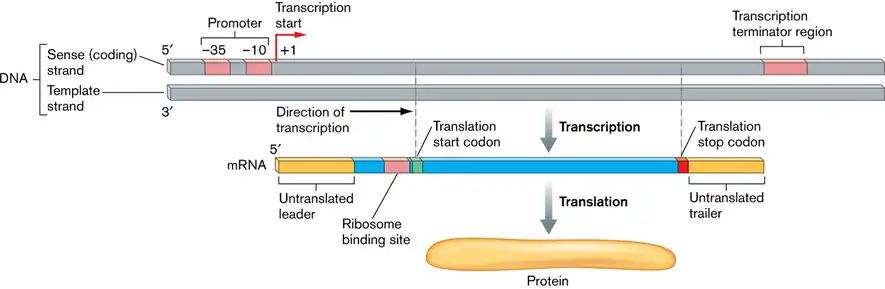
All aminoglycosides can cause either reversible or irreversible ototoxicity. Ototoxicity is more frequently observed in individuals who received the treatment for more than five days and those who have renal insufficiency.[5] The mechanism of aminoglycosides-induced ototoxicity is not well understood. It is thought that because cochlear cells are rich in mitochondria, these antibiotics may also target cochlear cells and cause their death.[8] Another hypothesis suggests that these drugs lead to the production of reactive oxygen species which generate oxidative stress and damage the inner ear.[9]
Glycopeptides
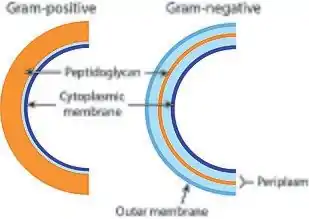
Glycopeptides are another class of antibiotics. Vancomycin is the class originator for the glycopeptides. Lipoglycopeptides are a subclass of glycopeptides and they are derived from the structure of vancomycin. Examples are telavancin and dalbavancin.[5]
Vancomycin and the lipoglycopeptides have slight differences in their mechanism of actions. Vancomycin inhibits cell wall synthesis of bacteria by preventing the cell wall component of bacteria, peptidoglycan, from elongating and cross-linking. With weakened peptidoglycan, the bacterial cell becomes susceptible to lysis.[7] Lipoglycopeptides, additionally, can increase the membrane permeability of the bacterial cell and disrupt the bacterial cell membrane potential.[7]
This class of antibiotics can be used to treat skin or joint infections, where gram-positive bacteria are the pathogens responsible. Vancomycin is also used as an initial empirical treatment agent of community-acquired bacterial meningitis in locations where penicillin-resistant S. pneumoniae is common.[10] This drug has other clinical uses, including endocarditis and respiratory tract infections caused by Methicillin-resistant Staphylococcus aureus (MRSA).
Case reports suggested that long-term use of vancomycin has been associated with ototoxicity.[11] However, there is no well-established causal link between vancomycin and ototoxicity. For instance, preclinical studies showed that vancomycin had a low risk of inducing ototoxicity.[2] Despite these findings, literature generally agreed that pre-existing hearing abnormalities, concomitant use of aminoglycosides and renal dysfunction are risk factors for vancomycin-induced ototoxicity.[12][13]
Chemotherapeutic agents
Chemotherapeutic agents are drugs that are used in chemotherapy for the treatment of cancer. Many of these agents are known to have the potential to cause hearing loss. Such agents include cisplatin and bleomycin.
Cisplatin
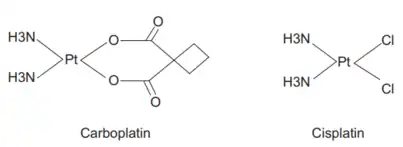
Cisplatin is known as a platinum coordination complex. Carboplatin and oxaliplatin also belong to platinum coordination complexes, but they are less commonly associated with ototoxicity. These agents are used in the treatment of ovarian, head and neck, bladder, lung and colon cancers. Cisplatin and other platinum coordination complexes work by reacting with various sites on DNA in mainly cancer cells in order to form cross-links. The formed DNA-platinum complexes inhibit replication and transcription, leading to miscoding and cell death.[5]
The mechanism of cisplatin in inducing ototoxicity is believed to involve the accumulation of reactive oxygen species, which exert cytotoxic effect on cochlear cells.[14] Some pharmacogenetics research have opened up new perspectives on the contributing factors of cisplatin-induced ototoxicity. They investigated several cancer-inducing genes and genetic polymorphisms. Results showed that some genes are associated with protective effect on ototoxicity, while others may show no effect or even increased effect on ototoxicity.[8]
Bleomycin
Bleomycin is one of the antitumour antibiotics and is a fermentation product of Streptomyces verticillus. It has a unique mechanism of action, making it an important agent in treating Hodgkin disease and testicular cancer. This unique chemotherapeutic agent causes oxidative damage to the nucleotides and leads to single- and double-stranded breaks in DNA. Excess breaks in DNA eventually causes cell death.[7] Few case reports have identified the development of ototoxicity in some elderly patients who were administered with bleomycin.[15] Since such cases are rare, the mechanisms behind have yet to be discovered.
Other examples of ototoxic medications
Non-steroidal anti-inflammatory drugs
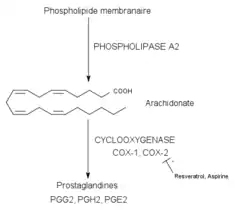
Non-steroidal anti-inflammatory drugs (NSAIDs) are one of the most recurrently used classes of drug clinically, indicated for anti-inflammatory, analgesic and antipyretic effects.[16] Examples include high-dose aspirin, ibuprofen and naproxen. Its therapeutic effect is achieved by inhibiting the activity of cyclooxygenase (COX), an enzyme mediating the biosynthesis of prostaglandins (PGs) and thromboxanes (TXAs). As this enzyme is inhibited, prostaglandin and thromboxane production is reduced, hence inhibiting the inflammatory response of pain and swelling caused by prostaglandin.[17]
Studies have proven that high-dose usage of aspirin can be associated with ototoxicity, manifesting reversible hearing loss and tinnitus.[18] The underlying mechanism is associated with a change in isolated cochlear outer hair cells (OHCs). Due to the COX inhibition, there is an increasing amount of leukotrienes in the inner ear. This leukotriene elevation leads to an alteration in the shape of isolated OHCs, thus disrupting their functions. Cochlear blood flow is eventually reduced, causing hearing impairment.[19]
Phosphodiesterase-5 inhibitors
Phosphodiesterase-5 (PDE-5) inhibitors are the first-line drugs indicated for erectile dysfunction (ED), implying the sustained impairment of erectile functioning, which may lead to unsatisfactory sexual performance.[20] Specific PDE-5 inhibitors are also approved for the treatment of benign prostatic hyperplasia, pulmonary hypertension and lower urinary tract symptoms.[21] Common drug examples include sildenafil, vardenafil and tadalafil. The enzyme PDE-5, found in the corpus cavernosum smooth muscle, is responsible for degrading cyclic guanosine monophosphate (cGMP) to 5-GMP. Inhibitors of this enzyme compete with cGMP for binding sites, which in turn increases the level of cGMP in smooth muscles. Through this mechanism, penis erection in male is eventually prolonged, resulting in a correction of ED. These drugs are known to cause headache, flushing and abnormal vision as their adverse effects.
PDE-5 inhibitors are also known for inducing sudden sensorineural hearing loss. It is mainly related to the obstruction and dysfunction of eustachian tubes which affects middle-ear pressure. Due to the high similarity in structure between the penile corpus cavernosum and nasal erectile tissue, PDE-5 inhibitors targeting on corpus cavernosum smooth muscle will also act on nasal erectile tissues, which are mainly located at the inferior turbinate, the middle turbinate and nasal septum. Hence, specific nasal areas may become congested. This mediates inflammatory responses in the eustachian tube which connects to the middle ear, causing an impact on middle ear pressure. Such events will eventually lead to sudden hearing loss.[22]
Antimalarials

Antimalarial drugs can be classified into several classes based on different mechanisms of action and effects, including quinoline-type drugs, naphthoquinone, antifolates, guanidine derived drugs, sesquiterpene lactones, etc. In particular, quinoline-type drugs are known to be ototoxic. Examples include chloroquine and hydroxychloroquine which are quinine-like. Apart from antimalarial effects, these drugs are also used in the treatment of other diseases such as dermatological, immunological, rheumatological, and severe infectious diseases.[23]
Various ototoxic effects are manifested by using antimalarial drugs, with dizziness being one of the most common one. Other effects include vestibular symptoms, hearing loss and tinnitus, which can appear to be both temporary or permanent.[23] Nonetheless, the underlying mechanisms of antimalarial-induced ototoxicity are still poorly understood. Studies have suggested that high doses of quinine have an impact on the central auditory pathway and auditory periphery, which leads to elongation and subsequent contraction of isolated OHCs in the cochlea. This structural alteration affects their functions and results in cochlear blood flow reduction.
Loop diuretics

Loop diuretics is a major class of diuretic drugs indicated for oedema due to heart failure, liver disease and kidney disease. It is also used for treating hypertension.[24] Common examples include furosemide, bumetanide and ethacrynic acid. Loop diuretics act on the thick ascending limb of the loop of Henle in the kidney nephrons. The major mechanism of ion reabsorption in the thick ascending limb is the active transport of ions through Na+-K+-2Cl− co-transporters (NKCCs). By binding to and inhibiting NKCCs at the apical membrane of the loop of Henle, the reabsorption of Na+, K+ and Cl- is impaired, contributing to a higher ion concentration in the lumen.[24] Hence, the ultimate effect of loop diuretics is a reduction in salt reabsorption and an increase in water excretion.
Loop diuretics-induced ototoxicity is suggested to be associated with their action on stria vascularis located on the lateral wall of the cochlea. This area is responsible for maintaining the balance of ions of endolymph. A high potassium concentration as well as a low sodium concentration should be maintained in the endolymph to allow cochlear hair cells to function normally.[25] As the inhibitory actions of loop diuretics will also target on NKCCs existing on membrane surfaces of stria vascularis marginal cells, there will be a disturbance on the ionic composition of endolymph.[26] Once the endocochlear potential cannot be maintained, hearing is temporarily impaired. It is noticed that the risk of ototoxicity caused by furosemide is much higher than that of bumetanide.
Monitoring and management of ototoxicity
Several approaches can be considered in managing patients who developed ototoxicity as an adverse reaction to the medications. Otoprotective agents may be given in order to prevent patients from developing ototoxicity. These agents potentially include D-methionine and L-N-acetylcysteine.[3] The use of D-methionine to protect against hearing loss induced by drugs like cisplatin and aminoglycosides is preliminarily supported by animal studies.[27] NMDA antagonists are also shown to limit aminoglycosides-induced ototoxicity.[28]
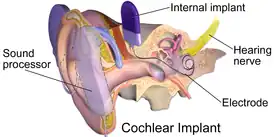
Restorative care which aims to regenerate hair cells that are damaged by ototoxic drugs can also be considered. For example, the infusion of neurotrophic factors (neurotrophin-3) was shown to produce otoprotective effects.[29] This protective agent was also found to be associated with survival of cochlear spiral ganglion neurones after hearing loss or deafness.[30]
Audiological management can be implemented, for example, providing hearing aids. In more seriously affected patients, cochlear implantation may be considered and discussed with the patient. It is also important for the healthcare team to educate affected patients and their family members on communication skills in order to minimise the impact on patients’ daily life.[3]
References
- Ganesan, Purushothaman; Schmiedge, Jason; Manchaiah, Vinaya; Swapna, Simham; Dhandayutham, Subhashini; Kothandaraman, Purushothaman Pavanjur (2018-04-10). "Ototoxicity: A Challenge in Diagnosis and Treatment". Journal of Audiology and Otology. 22 (2): 59–68. doi:10.7874/jao.2017.00360. ISSN 2384-1621. PMC 5894487. PMID 29471610.
- Lanvers-Kaminsky, C; Zehnhoff-Dinnesen, AG am; Parfitt, R; Ciarimboli, G (2017). "Drug-induced ototoxicity: Mechanisms, Pharmacogenetics, and protective strategies". Clinical Pharmacology & Therapeutics. 101 (4): 491–500. doi:10.1002/cpt.603. PMID 28002638. S2CID 41936433.
- "Guidelines from the Professional Board on Speech, Language and Hearing". Guidelines for Audiological management of patients on treatment that includes ototoxic medications. Health Professions Council of South Africa. 8 August 2023. pp. 6, 7, 15, 24–26. Retrieved 8 August 2023.
- Xie, Jing; Talaska, Andra E.; Schacht, Jochen (2011). "New developments in aminoglycoside therapy and ototoxicity". Hearing Research. 281 (1–2): 28–37. doi:10.1016/j.heares.2011.05.008. PMC 3169717. PMID 21640178.
- Goodman & Gilman's the pharmacological basis of therapeutics. Laurence L. Brunton, Randa Hilal-Dandan, Björn C. Knollmann (13 ed.). New York. 2018. ISBN 978-1-259-58473-2. OCLC 993810322.
{{cite book}}
: CS1 maint: location missing publisher (link) CS1 maint: others (link) - O’Sullivan, Mary E; Poitevin, Frédéric; Sierra, Raymond G; Gati, Cornelius; Dao, E Han; Rao, Yashas; Aksit, Fulya; Ciftci, Halilibrahim; Corsepius, Nicholas; Greenhouse, Robert; Hayes, Brandon (2018-10-12). "Aminoglycoside ribosome interactions reveal novel conformational states at ambient temperature". Nucleic Acids Research. 46 (18): 9793–9804. doi:10.1093/nar/gky693. ISSN 0305-1048. PMC 6182148. PMID 30113694.
- Basic & clinical pharmacology. Bertram G. Katzung, Todd W. Vanderah (15 ed.). [New York]. 2021. ISBN 978-1-260-45231-0. OCLC 1201912605.
{{cite book}}
: CS1 maint: location missing publisher (link) CS1 maint: others (link) - Lanvers-Kaminsky, Claudia; Ciarimboli, Giuliano (2017). "Pharmacogenetics of drug-induced ototoxicity caused by aminoglycosides and cisplatin". Pharmacogenomics. 18 (18): 1683–1695. doi:10.2217/pgs-2017-0125. ISSN 1462-2416. PMID 29173064.
- Wu, Wei-Jing; Sha, Su-Hua; Schacht, Jochen (2002). "Recent Advances in Understanding Aminoglycoside Ototoxicity and Its Prevention". Audiology and Neurotology. 7 (3): 171–174. doi:10.1159/000058305. ISSN 1420-3030. PMID 12053140. S2CID 32139933.
- Tunkel, Allan R.; Hartman, Barry J.; Kaplan, Sheldon L.; Kaufman, Bruce A.; Roos, Karen L.; Scheld, W. Michael; Whitley, Richard J. (2004-11-01). "Practice Guidelines for the Management of Bacterial Meningitis". Clinical Infectious Diseases. 39 (9): 1267–1284. doi:10.1086/425368. ISSN 1537-6591. PMID 15494903. S2CID 11833042.
- Humphrey, Clayton; Veve, Michael P.; Walker, Brian; Shorman, Mahmoud A. (2019-11-06). Crivellari, Martina (ed.). "Long-term vancomycin use had low risk of ototoxicity". PLOS ONE. 14 (11): e0224561. Bibcode:2019PLoSO..1424561H. doi:10.1371/journal.pone.0224561. ISSN 1932-6203. PMC 6834250. PMID 31693679.
- Rybak, Michael J.; Abate, Betty J.; Kang, S. Lena; Ruffing, Michael J.; Lerner, Stephen A.; Drusano, George L. (1999). "Prospective Evaluation of the Effect of an Aminoglycoside Dosing Regimen on Rates of Observed Nephrotoxicity and Ototoxicity". Antimicrobial Agents and Chemotherapy. 43 (7): 1549–1555. doi:10.1128/AAC.43.7.1549. ISSN 0066-4804. PMC 89322. PMID 10390201.
- Brummett, Robert E. (1993). "Ototoxicity of Vancomycin and Analogues". Otolaryngologic Clinics of North America. 26 (5): 821–828. doi:10.1016/S0030-6665(20)30769-6. PMID 8233491.
- Callejo, Angela; Sedó-Cabezón, Lara; Juan, Ivan; Llorens, Jordi (2015-07-15). "Cisplatin-Induced Ototoxicity: Effects, Mechanisms and Protection Strategies". Toxics. 3 (3): 268–293. doi:10.3390/toxics3030268. ISSN 2305-6304. PMC 5606684. PMID 29051464.
- "Bleomycin: Ototoxicity, dermatitis and nail disorders: case report". Reactions Weekly. 1579: 84. doi:10.1007/s40278-015-11045-x.
- Hoshino, Tomofumi; Tabuchi, Keiji; Hara, Akira (2010-04-27). "Effects of NSAIDs on the Inner Ear: Possible Involvement in Cochlear Protection". Pharmaceuticals. 3 (5): 1286–1295. doi:10.3390/ph3051286. ISSN 1424-8247. PMC 4033980. PMID 27713301.
- Vane, J. R.; Botting, R. M. (1998-12-03). "Anti-inflammatory drugs and their mechanism of action". Inflammation Research. 47: 78–87. doi:10.1007/s000110050284. ISSN 1023-3830. PMID 9831328. S2CID 1866687.
- Curhan, Sharon G.; Eavey, Roland; Shargorodsky, Josef; Curhan, Gary C. (2010). "Analgesic Use and the Risk of Hearing Loss in Men". The American Journal of Medicine. 123 (3): 231–237. doi:10.1016/j.amjmed.2009.08.006. PMC 2831770. PMID 20193831.
- Jung, Timothy T. K.; Kim, John P. S.; Bunn, Jeffrey; Davamony, David; Duncan, John; Fletcher, William H. (1997). "Effect of Leukotriene Inhibitor on Salicylate Induced Morphologic Changes of Isolated Cochlear Outer Hair Cells". Acta Oto-Laryngologica. 117 (2): 258–264. doi:10.3109/00016489709117783. ISSN 0001-6489. PMID 9105462.
- Huang, Sharon A.; Lie, Janette D. (2013). "Phosphodiesterase-5 (PDE5) Inhibitors In the Management of Erectile Dysfunction". P & T: A Peer-Reviewed Journal for Formulary Management. 38 (7): 407–419. ISSN 1052-1372. PMC 3776492. PMID 24049429.
- Tinel, Hanna; Stelte-Ludwig, Beatrix; Hütter, Joachim; Sandner, Peter (2006). "Pre-clinical evidence for the use of phosphodiesterase-5 inhibitors for treating benign prostatic hyperplasia and lower urinary tract symptoms". BJU International. 98 (6): 1259–1263. doi:10.1111/j.1464-410X.2006.06501.x. ISSN 1464-4096. PMID 16956354. S2CID 221531271.
- Okuyucu, S; Guven, O E; Akoglu, E; Uçar, E; Dagli, S (2009). "Effect of phosphodiesterase-5 inhibitor on hearing". The Journal of Laryngology & Otology. 123 (7): 718–722. doi:10.1017/S002221510900423X. ISSN 0022-2151. PMID 19134242. S2CID 5583002.
- Jozefowicz-Korczynska, Magdalena; Pajor, Anna; Lucas Grzelczyk, Weronika (2021). "The Ototoxicity of Antimalarial Drugs-A State of the Art Review". Frontiers in Neurology. 12: 661740. doi:10.3389/fneur.2021.661740. ISSN 1664-2295. PMC 8093564. PMID 33959089.
- Sarafidis, Pantelis A; Georgianos, Panagiotis I; Lasaridis, Anastasios N (2010). "Diuretics in clinical practice. Part I: mechanisms of action, pharmacological effects and clinical indications of diuretic compounds". Expert Opinion on Drug Safety. 9 (2): 243–257. doi:10.1517/14740330903499240. ISSN 1474-0338. PMID 20095917. S2CID 7303716.
- Hopkins, Kathryn (2015), "Deafness in cochlear and auditory nerve disorders", The Human Auditory System - Fundamental Organization and Clinical Disorders, Handbook of Clinical Neurology, vol. 129, Elsevier, pp. 479–494, doi:10.1016/b978-0-444-62630-1.00027-5, ISBN 978-0-444-62630-1, PMID 25726286, retrieved 2022-03-15
- Ding, Dalian; Liu, Hong; Qi, Weidong; Jiang, Haiyan; Li, Yongqi; Wu, Xuewen; Sun, Hong; Gross, Kenneth; Salvi, Richard (2016). "Ototoxic effects and mechanisms of loop diuretics". Journal of Otology. 11 (4): 145–156. doi:10.1016/j.joto.2016.10.001. PMC 6002634. PMID 29937824.
- Campbell, Kathleen C.M.; Meech, Robert P.; Klemens, James J.; Gerberi, Michael T.; Dyrstad, Sara S.W.; Larsen, Deb L.; Mitchell, Diana L.; El-Azizi, Mohammed; Verhulst, Steven J.; Hughes, Larry F. (2007). "Prevention of noise- and drug-induced hearing loss with d-methionine". Hearing Research. 226 (1–2): 92–103. doi:10.1016/j.heares.2006.11.012. PMID 17224251. S2CID 24953862.
- Basile, Anthony S.; Huang, Jer-Min; Xie, Chen; Webster, Douglas; Berlin, Charles; Skolnick, Phil (1996). "N–Methyl–D–aspartate antagonists limit aminoglycoside antibiotic–induced hearing loss". Nature Medicine. 2 (12): 1338–1343. doi:10.1038/nm1296-1338. ISSN 1078-8956. PMID 8946832. S2CID 30861122.
- Ernfors, Patrik; Li Duan, Mao; Elshamy, Wael M.; Canlon, Barbara (1996). "Protection of auditory neurons from aminoglycoside toxicity by neurotrophin-3". Nature Medicine. 2 (4): 463–467. doi:10.1038/nm0496-463. ISSN 1078-8956. PMID 8597959. S2CID 22927156.
- Leake, Patricia A.; Akil, Omar; Lang, Hainan (2020). "Neurotrophin gene therapy to promote survival of spiral ganglion neurons after deafness". Hearing Research. 394: 107955. doi:10.1016/j.heares.2020.107955. PMC 7660539. PMID 32331858.