Oxidative coupling of phenols
Oxidative coupling of phenols is a chemical reaction wherein two phenolic compounds are coupled via an oxidative process. Oxidative phenol couplings are often catalyzed by transition metal complexes including V, Cr, Mn, Cu, Fe, among others. Such reactions often form C–C, or C–O bonds between the coupling partners and can be employed as either homo- or cross-couplings.
History
The first example of an oxidative phenol coupling in synthetic chemistry can be traced to Julius Löwe’s 1868 synthesis of ellagic acid, accomplished by heating gallic acid with arsenic acid.[1]
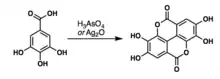
General challenges
Oxidative phenol couplings are particularly attractive and simplifying retrosynthetic disconnections for their atom economy and lack of pre-functionalized starting materials often seen in traditional redox-neutral cross-couplings. In practice, however, challenges exist in their successful implementation in synthesis.
Oxidative phenol couplings often suffer from over-oxidation as a potential side product. This is a common occurrence if the product formed by the initial coupling is more oxidizable (has a lower oxidation potential) than the starting material. In such cases, the catalyst can be quenched or poisoned by engaging in off-cycle redox processes with the product. Additionally, the product may oxidize further, giving way to higher-order oligomers.
Selectivity issues may arise during oxidative phenol couplings between C–C coupled and C–O coupled products.[2] Moreover, stereoselectivity is an important consideration if the resulting biphenol compound displays axial chirality or atropoisomerism. Selectivity between homo- and hetero-coupled products must be considered, and can often be addressed through transition-metal catalysis.
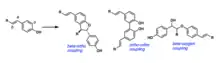
Mechanistic manifolds
Oxidative phenol couplings can occur through either inner sphere or outer sphere processes. In inner sphere processes, the substrate binds directly to a transition metal. Oxidation to the phenol occurs via electron transfer or hydrogen atom abstraction from the metal. The resulting reactive intermediate can engage in downstream chemical processes which can occur via either coordinated (inner-sphere) or non-coordinated coupling partners.
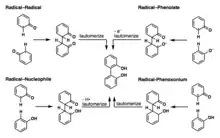
In general, 4 groups of mechanisms have been observed:
Radical-radical
Two oxidized phenols undergo radical recombination followed by tautomerization to furnish the product. This process is often favored when metal-stabilized radicals are formed., and product selectivity depends on the spin density of radicals at different positions of the arene.
Radical-nucleophile
Phenol radical reacts directly with another equivalent of phenol, followed by hydrogen atom abstraction and tautomerization.
Radical-phenolate
Electron transfer occurs to one phenol and the resulting radical reacts with an equivalent of phenoxide. Subsequent single electron oxidation and tautomerization yields the product.
Phenol-Phenoxonium
Two-electron oxidation of phenol followed by reaction with another equivalent of phenol and tautomerization affords the product.
Homocouplings
Site selectivity in functionalized phenols is often empirical, but can be supported by calculations of positions containing the highest radical character and least steric hindrance. In select cases, however radical localization preferences can be overcome by kinetic control guided by steric hindrance of the positions or thermodynamic control guided by reversible couplings followed by irreversible rearomatization.
In general, couplings where metal catalysts are not involved in the coupling (including photochemical processes) often proceed via the radical-phenol mechanism and display substrate-controlled selectivity.[3][4][5]
Though select examples of unsymmetrical homocouplings are known, they are notoriously challenging to design and are often arrived at empirically.
Enantioselective asymmetric phenol oxidative couplings are not well-established or general yet, however there exist reports leveraging asymmetric vanadium catalysts to enantioselectively homocouple phenols. In contrast, much progress has been made in asymmetric 2-napthol couplings using Ru, Cu, V, and Fe catalysts,[6] which have had a large impact on the development of BINAP-type ligands used asymmetric catalysis.
Intramolecular phenol couplings
Though early examples of intramolecular oxidative phenol couplings were discovered, little progress has been made in this area. The most well-studied examples of such transformations are those yielding spirocyclic phenol-dienone coupled products. The coupling partners in an intramolecular coupling must approach in a near-parallel arrangement to allow for orbital overlap;[7] these stringent geometric restraints on pre-cyclized compounds often render the process sluggish, if possible.[8]
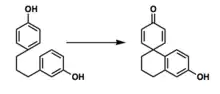
C–O couplings
Though a mechanistic possibility when phenols oxidized, methodologies for selective C–O coupling of phenols are limited and couplings remain largely substrate dependent.[9] In many cases, selective C–O coupling can only be achieved if all ortho and para-positions on the arene are blocked.[10] Poor C–O coupling selectivity is likely due to the lack of radical spin-density on oxygen after phenol oxidation, resulting in kinetic trapping of C–C coupling products.
Nonphenolic arene couplings
Oxidative couplings have also been studied between phenols and nonphenolic compounds including anilines, beta-ketoesters/malonates/malononitriles, electron-rich arenes, olefins, and other functional groups.[11]
References
- Löwe, Julius (1868). "Über die Bildung von Ellagsäure aus Gallussäure" [On the synthesis of ellagic acid from gallic acid]". Zeitschrift für Chemie. 4: 603.
- Neuhaus, William C.; Jemison, Adriana L.; Kozlowski, Marisa C. (6 October 2021). "Oxidative dehydrogenative couplings of alkenyl phenols". Organic & Biomolecular Chemistry. 19 (38): 8205–8226. doi:10.1039/D1OB01040A. ISSN 1477-0539. PMC 8497443. PMID 34522924.
- Niederer, Kyle A.; Gilmartin, Philip H.; Kozlowski, Marisa C. (18 December 2020). "Oxidative Photocatalytic Homo- and Cross-Coupling of Phenols: Nonenzymatic, Catalytic Method for Coupling Tyrosine". ACS Catalysis. 10 (24): 14615–14623. doi:10.1021/acscatal.0c04515. ISSN 2155-5435. PMC 8078885. PMID 33927912.
- Nakajima, Makoto; Miyoshi, Irie; Kanayama, Kumiko; Hashimoto, Shun-ichi; Noji, Masahiro; Koga, Kenji (1 April 1999). "Enantioselective Synthesis of Binaphthol Derivatives by Oxidative Coupling of Naphthol Derivatives Catalyzed by Chiral Diamine·Copper Complexes". The Journal of Organic Chemistry. 64 (7): 2264–2271. doi:10.1021/jo981808t. ISSN 0022-3263.
- Li, Xiaolin; Yang, Jaemoon; Kozlowski, Marisa C. (1 April 2001). "Enantioselective Oxidative Biaryl Coupling Reactions Catalyzed by 1,5-Diazadecalin Metal Complexes". Organic Letters. 3 (8): 1137–1140. doi:10.1021/ol015595x. ISSN 1523-7060. PMID 11348178.
- Nakajima, Makoto; Kanayama, Kumiko; Miyoshi, Irie; Hashimoto, Shun-ichi (25 December 1995). "Catalytic asymmetric synthesis of binaphthol derivatives by aerobic oxidative coupling of 3-hydroxy-2-naphthoates with chiral diamine-copper complex". Tetrahedron Letters. 36 (52): 9519–9520. doi:10.1016/0040-4039(95)02063-2. ISSN 0040-4039.
- Armstrong, David R.; Cameron, Colin; Nonhebel, Derek C.; Perkins, Peter G. (1 January 1983). "Oxidative coupling of phenols. Part 8. A theoretical study of the coupling of phenoxyl radicals". Journal of the Chemical Society, Perkin Transactions 2 (5): 575–579. doi:10.1039/P29830000575. ISSN 1364-5471.
- Nieves-Quinones, Yexenia; Paniak, Thomas J.; Lee, Young Eun; Kim, Sun Min; Tcyrulnikov, Sergei; Kozlowski, Marisa C. (26 June 2019). "Chromium-Salen Catalyzed Cross-Coupling of Phenols: Mechanism and Origin of the Selectivity". Journal of the American Chemical Society. 141 (25): 10016–10032. doi:10.1021/jacs.9b03890. ISSN 0002-7863. PMC 6628261. PMID 31125210.
- Tanaka, Kumpei; Gotoh, Hiroaki (19 July 2019). "Development of the radical C–O coupling reaction of phenols toward the synthesis of natural products comprising a diaryl ether skeleton". Tetrahedron. 75 (29): 3875–3885. doi:10.1016/j.tet.2019.05.035. ISSN 0040-4020. S2CID 191193995.
- Neuhaus, William C.; Kozlowski, Marisa C. (11 May 2020). "Total Synthesis of Pyrolaside B: Phenol Trimerization through Sequenced Oxidative C−C and C−O Coupling". Angewandte Chemie International Edition. 59 (20): 7842–7847. doi:10.1002/anie.201915654. ISSN 1433-7851. PMC 7200290. PMID 32026544.
- Wu, Jingze; Kozlowski, Marisa C. (3 June 2022). "Catalytic Oxidative Coupling of Phenols and Related Compounds". ACS Catalysis. 12 (11): 6532–6549. doi:10.1021/acscatal.2c00318. ISSN 2155-5435. PMC 9345132. PMID 35928569.