Two-photon physics
Two-photon physics, also called gamma–gamma physics, is a branch of particle physics that describes the interactions between two photons. Normally, beams of light pass through each other unperturbed. Inside an optical material, and if the intensity of the beams is high enough, the beams may affect each other through a variety of non-linear effects. In pure vacuum, some weak scattering of light by light exists as well. Also, above some threshold of this center-of-mass energy of the system of the two photons, matter can be created.
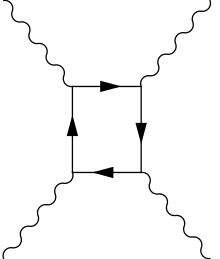
Astronomy
Cosmological/intergalactic gamma rays
Photon–photon interactions limit the spectrum of observed gamma-ray photons at moderate cosmological distances to a photon energy below around 20 GeV, that is, to a wavelength of greater than approximately 6.2×10−11 m. This limit reaches up to around 20 TeV at merely intergalactic distances. [1] An analogy would be light traveling through a fog: At near distances a light source is more clearly visible than can at long distances due to the scattering of light by fog particles. Similarly, the further a gamma-ray travels through the universe, the more likely it is to be scattered by an interaction with a low energy photon from the extragalactic background light.
At those energies and distances, very high energy gamma-ray photons have a significant probability of a photon-photon interaction with a low energy background photon from the extragalactic background light resulting in either the creation of particle-antiparticle pairs via direct pair production or (less often) by photon-photon scattering events that lower the incident photon energies. This renders the universe effectively opaque to very high energy photons at intergalactic to cosmological distances.
Experiments
Two-photon physics can be studied with high-energy particle accelerators, where the accelerated particles are not the photons themselves but charged particles that will radiate photons. The most significant studies so far were performed at the Large Electron–Positron Collider (LEP) at CERN. If the transverse momentum transfer and thus the deflection is large, one or both electrons can be detected; this is called tagging. The other particles that are created in the interaction are tracked by large detectors to reconstruct the physics of the interaction.
Frequently, photon-photon interactions will be studied via ultraperipheral collisions (UPCs) of heavy ions, such as gold or lead. These are collisions in which the colliding nuclei do not touch each other; i.e., the impact parameter is larger than the sum of the radii of the nuclei. The strong interaction between the quarks composing the nuclei is thus greatly suppressed, making the weaker electromagnetic interaction much more visible. In UPCs, because the ions are heavily charged, it is possible to have two independent interactions between a single ion pair, such as production of two electron-positron pairs. UPCs are studied with the STARlight simulation code.
Light-by-light scattering can be studied using the strong electromagnetic fields of the hadrons collided at the LHC,[2][3] it has first been seen in 2016 by the ATLAS collaboration[4][5] and was then confirmed by the CMS collaboration.[6], including at high two-photon energies.[7] The best previous constraint on the elastic photon–photon scattering cross section was set by PVLAS, which reported an upper limit far above the level predicted by the Standard Model.[8] Observation of a cross section larger than that predicted by the Standard Model could signify new physics such as axions, the search of which is the primary goal of PVLAS and several similar experiments.
Processes
From quantum electrodynamics it can be found that photons cannot couple directly to each other and a fermionic field according to the Landau-Yang theorem[9] since they carry no charge and no 2 fermion + 2 boson vertex exists due to requirements of renormalizability, but they can interact through higher-order processes or couple directly to each other in a vertex with an additional two W bosons: a photon can, within the bounds of the uncertainty principle, fluctuate into a virtual charged fermion–antifermion pair, to either of which the other photon can couple. This fermion pair can be leptons or quarks. Thus, two-photon physics experiments can be used as ways to study the photon structure, or, somewhat metaphorically, what is "inside" the photon.
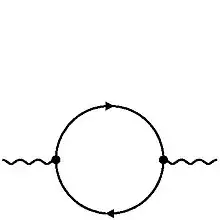
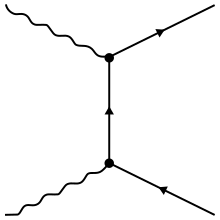
There are three interaction processes:
- Direct or pointlike: The photon couples directly to a quark inside the target photon.[10] If a lepton–antilepton pair is created, this process involves only quantum electrodynamics (QED), but if a quark–antiquark pair is created, it involves both QED and perturbative quantum chromodynamics (QCD).[11][12][13]
The intrinsic quark content of the photon is described by the photon structure function, experimentally analyzed in deep-inelastic electron–photon scattering.[14][15]
- Single resolved: The quark pair of the target photon form a vector meson. The probing photon couples to a constituent of this meson.
- Double resolved: Both target and probe photon have formed a vector meson. This results in an interaction between two hadrons.
For the latter two cases, the scale of the interaction is such as the strong coupling constant is large. This is called vector meson dominance (VMD) and has to be modelled in non-perturbative QCD.
See also
- Channelling radiation has been considered as a method to generate polarized high energy photon beams for gamma–gamma colliders.
- Matter creation
- Pair production
- Delbrück scattering
- Breit–Wheeler process
References
- Franceschini, Alberto (14 May 2021). "Photon–Photon Interactions and the Opacity of the Universe in Gamma Rays". Universe. 7 (5). 146. Bibcode:2021Univ....7..146F. doi:10.3390/universe7050146.
{{cite journal}}
: CS1 maint: date and year (link) - d'Enterria, David; da Silveira, Gustavo G. (22 August 2013). "Observing Light-by-Light Scattering at the Large Hadron Collider". Physical Review Letters. American Physical Society (APS). 111 (8): 080405. arXiv:1305.7142. Bibcode:2013PhRvL.111h0405D. doi:10.1103/physrevlett.111.080405. ISSN 0031-9007. PMID 24010419. S2CID 43797550.
- Michael Schirber (22 Aug 2013). "Synopsis: Spotlight on Photon-Photon Scattering". Physical Review Letters. 111 (8): 080405. arXiv:1305.7142. Bibcode:2013PhRvL.111h0405D. doi:10.1103/PhysRevLett.111.080405. PMID 24010419. S2CID 43797550.
- "ATLAS spots light-by-light scattering". CERN Courier. 11 Nov 2016. Retrieved 27 May 2019.
- ATLAS Collaboration: Light-by-light scattering in ultra-peripheral Pb+Pb collisions at √sNN=5.02 TeV with the ATLAS detector at the LHC
- Collaboration, CMS (2019). "Evidence for light-by-light scattering and searches for axion-like particles in ultraperipheral PbPb collisions at = 5.02 TeV". Phys. Lett. B. 797: 134826. arXiv:1810.04602. doi:10.1016/j.physletb.2019.134826. S2CID 201698459.
- CMS Collaboration†; TOTEM Collaboration‡; Tumasyan, A.; Adam, W.; Bergauer, T.; Dragicevic, M.; Erö, J.; Escalante Del Valle, A.; Frühwirth, R.; Jeitler, M.; Krammer, N.; Lechner, L.; Liko, D.; Mikulec, I.; Pitters, F. M. (2022-06-28). "First Search for Exclusive Diphoton Production at High Mass with Tagged Protons in Proton-Proton Collisions at $\sqrt{s}=13\text{ }\text{ }\mathrm{TeV}$". Physical Review Letters. 129 (1): 011801. doi:10.1103/PhysRevLett.129.011801.
- Zavattini, G.; Gastaldi, U.; Pengo, R.; Ruoso, G.; Valle, F. Della; Milotti, E. (20 June 2012). "Measuring the magnetic birefringence of vacuum: the PVLAS experiment". International Journal of Modern Physics A. World Scientific Pub Co Pte Lt. 27 (15): 1260017. arXiv:1201.2309. Bibcode:2012IJMPA..2760017Z. doi:10.1142/s0217751x12600172. ISSN 0217-751X. S2CID 119248772.
- Igor P. Ivanov1, Valeriy G. Serbo2,3, Pengming Zhang4,5, Fate of the Landau-Yang theorem for twisted photons, https://arxiv.org/pdf/1904.12110.pdf "What is actually forbidden is production of a spin-1 particle by such a photon pair"
- Walsh, T.F.; Zerwas, P. (1973). "Two-photon processes in the parton model". Physics Letters B. Elsevier BV. 44 (2): 195–198. Bibcode:1973PhLB...44..195W. doi:10.1016/0370-2693(73)90520-0. ISSN 0370-2693.
- Witten, Edward (1977). "Anomalous cross section for photon-photon scattering in gauge theories". Nuclear Physics B. Elsevier BV. 120 (2): 189–202. Bibcode:1977NuPhB.120..189W. doi:10.1016/0550-3213(77)90038-4. ISSN 0550-3213.
- Bardeen, William A.; Buras, Andrzej J. (1 June 1979). "Higher-order asymptotic-freedom corrections to photon-photon scattering". Physical Review D. American Physical Society (APS). 20 (1): 166–178. Bibcode:1979PhRvD..20..166B. doi:10.1103/physrevd.20.166. ISSN 0556-2821.
- Bardeen, William A.; Buras, Andrzej J. (1 March 1980). "Erratum: Higher-order asymptotic-freedom corrections to photon-photon scattering". Physical Review D. American Physical Society (APS). 21 (7): 2041. Bibcode:1980PhRvD..21.2041B. doi:10.1103/physrevd.21.2041. ISSN 0556-2821.
- Achard, P.; et al. (L3 collaboration) (2005). "Measurement of the photon structure function F2γ with the L3 detector at LEP". Physics Letters B. 622 (3–4): 249–264. arXiv:hep-ex/0507042. Bibcode:2005PhLB..622..249A. doi:10.1016/j.physletb.2005.07.028. ISSN 0370-2693. S2CID 119346514.
- Nisius, Richard (2000). "The photon structure from deep inelastic electron–photon scattering". Physics Reports. 332 (4–6): 165–317. arXiv:hep-ex/9912049. Bibcode:2000PhR...332..165N. doi:10.1016/s0370-1573(99)00115-5. ISSN 0370-1573. S2CID 119437227.