Titanate nanosheet
Titanate (IV) nanosheets (TiNSs) have a 2D structure where TiO6 octahedra are edge-linked in a lepidocrocite-type 2D lattice[1] with chemical formula HxTi2—x/4☐x/4O4 ⦁ H2O (x~0.7; ☐, vacancy).[2] Titanate nanosheets may be regarded as sheets with molecular thickness and infinite planar dimensions. TiNSs are typically formed via liquid-phase exfoliation of protonic titanate. In inorganic layered materials, individual layers are bound to each other by van der Waals interactions if they are neutral, and additional Coulomb interactions if they are composed of oppositely charged layers. Through liquid-phase exfoliation, these individual sheets of layered materials can be efficiently separated using an appropriate solvent, creating single-layer colloidal suspensions.[3] Solvents must have an interaction energy with the layers that is greater than the interaction energy between two layers.[3] In situ X-Ray diffraction data indicates that TiNSs can be treated as macromolecules with a sufficient amount of solvent in between layers so that they behave as individual sheets.[2]
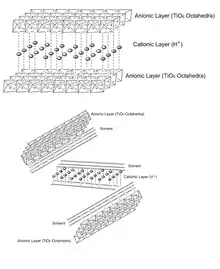

Properties
Unilamellar TiNSs have a number of unique properties, and are said to combine those of conventional titanate and titania. Structurally, they are infinite ultrathin (~0.75 nm) 2D sheets with a high density of negative surface charges originating from the oxygen atoms at the corners of the adjoint octahedrons[4] . TiNSs may balance this anionic charge by inserting a counterionic layer between the two sheets either via layering or in aqueous solution. This electric double layer gives the material flexible interlayer distances,[5] high cation exchange capacity,[5] and excellent dielectric capabilities.[1]
Typically, titanium oxide suffers from oxygen vacancies, which diminish its potential as capacitors due to vacancies acting as high-leakage paths and charge carrier traps,[1] however, TiNSs possess Ti vacancies, which promote channels for electron transfer.[1] When Ti vacancies are present, the effective charge felt by electrons on oxygen atoms reduces and allows less hindered electron motion.[6]
Applications

TiNSs can act as highly efficient adsorbents and photocatalysts due to their two-dimensional geometry and structure. This phenomenon can be exploited for several applications, including the removal of metal ions and dyes from water systems.[5] Further, TiNSs potential as an electrocatalyst may enhance fuel cell efficiency during fuel oxidation.[7] Similarly, intercalated myoglobin is proven to be an efficient catalyst for H2O2.[8]
TiNSs may also be used for immobilizing biomolecules. When a monolayer of hemoglobin is intercalated into TiNSs, the electron transfer between the active sites of the protein and the electrodes is amplified, and electrocatalytic activity for O2 reduction increases. In addition, heterostructured nanosheets of Fe3O4-Na2Ti3O7 can be used for protein separation. When placed in the aqueous environment at pH 6, positively charged hemoglobin binds to the nanosheets, whereas negative albumin can be detected in the supernatant.[9]
Perhaps the most interesting application of TiNSs is in the development of a material dominated by electrostatically repulsive interactions. TiNSs exhibit maximum electrostatic repulsion when they are aligned cofacially. To create the hydrogel based on this, a solution of TiNSs is placed in a strong magnetic field where repulsive forces induce a quasi-crystalline structure. Upon irradiation with UV-light, the solution polymerizes and creates cross-linked network, which is non-covalently attached to the TiNSs.[4] This creates a composite that resists orthogonally applied compressive forces, but easily deforms due to shear forces.[10] TiNSs solutions of this sort may be used as an anti-vibration or vibration-isolating material[11] and in the design of artificial cartilage.[4]
Titanate nanosheets can also be aligned within polymer, parallel to the surface of the substrate by simple drop casting.[12] The intercalation of polymer and orientation of nanosheets were studied by small-angle X-ray scattering (SAXS) using in-plane and symmetrical scan. SAXS mapping indicated homogeneous alignment of titanate nanosheets within polymer. The mechanical reinforcement of polyamic acid using titanate nanosheets matched with Halpin-Tsai model, which is a composite model that assume the filler is in aligned position.
References
- Osada, Minoru; Sasaki, Takayoshi (2011-10-14). "Two-Dimensional Dielectric Nanosheets: Novel Nanoelectronics From Nanocrystal Building Blocks". Advanced Materials. Wiley. 24 (2): 210–228. doi:10.1002/adma.201103241. ISSN 0935-9648. PMID 21997712.
- Sasaki, Takayoshi; Watanabe, Mamoru; Hashizume, Hideo; Yamada, Hirohisa; Nakazawa, Hiromoto (1996-01-01). "Macromolecule-like Aspects for a Colloidal Suspension of an Exfoliated Titanate. Pairwise Association of Nanosheets and Dynamic Reassembling Process Initiated from It". Journal of the American Chemical Society. American Chemical Society (ACS). 118 (35): 8329–8335. doi:10.1021/ja960073b. ISSN 0002-7863.
- M. Brown (2011-02-03). "Exfoliating layered materials". Retrieved 2021-12-18.
- Liu, Mingjie; Ishida, Yasuhiro; Ebina, Yasuo; Sasaki, Takayoshi; Hikima, Takaaki; Takata, Masaki; Aida, Takuzo (2014-12-31). "An anisotropic hydrogel with electrostatic repulsion between cofacially aligned nanosheets". Nature. Springer Science and Business Media LLC. 517 (7532): 68–72. doi:10.1038/nature14060. ISSN 0028-0836. PMID 25557713.
- Huang, Jiquan; Cao, Yongge; Deng, Zhonghua; Tong, Hao (2011). "Formation of titanate nanostructures under different NaOH concentration and their application in wastewater treatment". Journal of Solid State Chemistry. Elsevier BV. 184 (3): 712–719. doi:10.1016/j.jssc.2011.01.023. ISSN 0022-4596.
- Ohwada, Megumi; Kimoto, Koji; Mizoguchi, Teruyasu; Ebina, Yasuo; Sasaki, Takayoshi (2013-09-30). "Atomic structure of titania nanosheet with vacancies". Scientific Reports. Springer Science and Business Media LLC. 3 (1). doi:10.1038/srep02801. ISSN 2045-2322. PMC 3786289.
- Bavykin, Dmitry V.; Walsh, Frank C. (2009). "Elongated Titanate Nanostructures and Their Applications". European Journal of Inorganic Chemistry. Wiley. 2009 (8): 977–997. doi:10.1002/ejic.200801122. ISSN 1434-1948.
- Zhang, L.; Zhang, Q.; Li, J. (2007-07-12). "Layered Titanate Nanosheets Intercalated with Myoglobin for Direct Electrochemistry". Advanced Functional Materials. Wiley. 17 (12): 1958–1965. doi:10.1002/adfm.200600991. ISSN 1616-301X.
- Zhou, Qinhua; Lu, Zhufeng; Cao, Xuebo (2014). "Heterostructured magnetite-titanate nanosheets for prompt charge selective binding and magnetic separation of mixed proteins". Journal of Colloid and Interface Science. Elsevier BV. 415: 48–56. doi:10.1016/j.jcis.2013.10.012. ISSN 0021-9797. PMID 24267329.
- RIKEN (30 December 2014). "A repulsive material: New hydrogel dominated by electrostatic repulsion". ScienceDaily.
- Ladegaard Skov, Anne (2014-12-31). "Like cartilage, but simpler". Nature. Springer Science and Business Media LLC. 517 (7532): 25–26. doi:10.1038/517025a. ISSN 0028-0836. PMID 25557709.
- Harito, Christian; Bavykin, Dmitry V.; Light, Mark E. & Walsh, Frank C. (2017). "Titanate nanotubes and nanosheets as a mechanical reinforcement of water-soluble polyamic acid: Experimental and theoretical studies" (PDF). Composites Part B: Engineering. 124: 54–63. doi:10.1016/j.compositesb.2017.05.051.