The resistivity of all materials depends on temperature. Some materials can become superconductors (zero resistivity) at very low temperatures (see ). Conversely, the resistivity of conductors increases with increasing temperature. Since the atoms vibrate more rapidly and over larger distances at higher temperatures, the electrons moving through a metal, for example, create more collisions, effectively making the resistivity higher. Over relatively small temperature changes (about 100ºC or less), resistivity ρ varies with temperature change ΔT as expressed in the following equation:
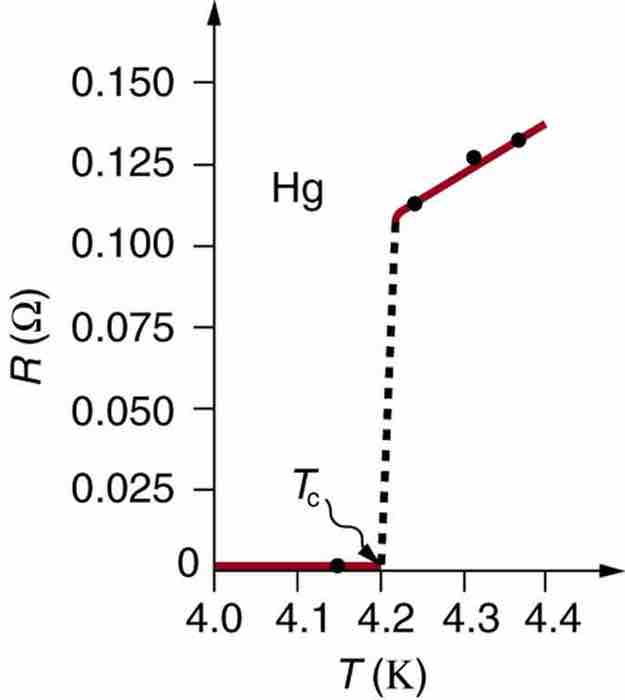
Resistance of a sample of mercury
The resistance of a sample of mercury is zero at very low temperatures—it is a superconductor up to about 4.2 K. Above that critical temperature, its resistance makes a sudden jump and then increases nearly linearly with temperature.
where ρ0 is the original resistivity and α is the temperature coefficient of resistivity. For larger temperature changes, α may vary, or a nonlinear equation may be needed to find ρ. For this reason it is usual to specify a suffix for the temperature at which the substance was measured (such as α15) and the relationship only holds in a range of temperatures around the reference. Note that α is positive for metals, meaning their resistivity increases with temperature. The temperature coefficient is typically +3×10−3 K−1 to +6×10−3 K−1 for metals near room temperature. Some alloys have been developed specifically to have a small temperature dependence. Manganin (made of copper, manganese and nickel), for example, has α close to zero, so its resistivity varies only slightly with temperature. This is useful for making a temperature-independent resistance standard, for example.
Note also that α is negative for semiconductors, meaning that their resistivity decreases with increasing temperature. They become better conductors at higher temperature because increased thermal agitation increases the number of free charges available to carry current. This property of decreasing ρ with temperature is also related to the type and amount of impurities present in the semiconductors.
The resistance of an object also depends on temperature, since R0 is directly proportional to ρ. For a cylinder we know R=ρL/A, so if L and A do not change greatly with temperature, R will have the same temperature dependence as ρ. (Examination of the coefficients of linear expansion shows them to be about two orders of magnitude less than typical temperature coefficients of resistivity, and so the effect of temperature on L and A is about two orders of magnitude less than on ρ. ) Thus,
is the temperature dependence of the resistance of an object, where R0 is the original resistance and R is the resistance after a temperature change T. Numerous thermometers are based on the effect of temperature on resistance (see ). One of the most common is the thermistor, a semiconductor crystal with a strong temperature dependence, the resistance of which is measured to obtain its temperature. The device is small so it quickly comes into thermal equilibrium with the part of a person it touches.
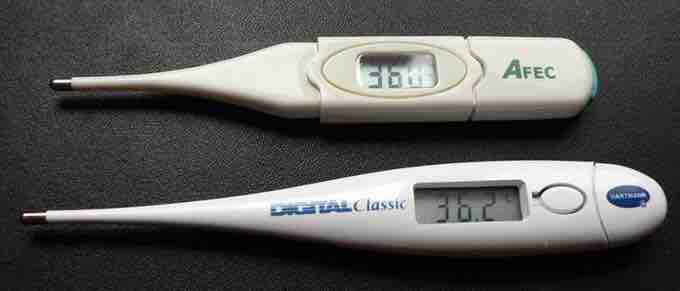
Thermometers
These familiar thermometers are based on the automated measurement of a thermistor's temperature-dependent resistance.