The de Broglie hypothesis, formulated in 1924, predicts that particles should also behave as waves. The wavelength of an electron is given by the de Broglie equation
From the work by Planck (black body radiation) and Einstein (photoelectric effect), physicists understood that electromagnetic waves sometimes behaved like particles. De Broglie's hypothesis is complementary to this idea: particles should also show wave-like properties such as diffraction or interference. De Broglie's formula was confirmed three years later for electrons (which have a rest-mass) with the observation of electron diffraction in two independent experiments. George Paget Thomson passed a beam of electrons through a thin metal film and observed the predicted interference patterns. Clinton Joseph Davisson and Lester Halbert Germerguided their beam through a crystalline grid to observe diffraction patterns.
X-ray diffraction is a commonly used tool in materials research. Thanks to the wave-particle duality, matter wave diffraction can also be used for this purpose. The electron, which is easy to produce and manipulate, is a common choice. A neutron is another particle of choice. Due to the different kinds of interactions involved in the diffraction processes, the three types of radiation (X-ray, electron, neutron) are suitable for different kinds of studies.
Electron diffraction is most frequently used in solid state physics and chemistry to study the crystalline structure of solids. Experiments are usually performed using a transmission electron microscope or a scanning electron microscope. In these instruments, electrons are accelerated by an electrostatic potential in order to gain the desired energy and, thus, wavelength before they interact with the sample to be studied. The periodic structure of a crystalline solid acts as a diffraction grating, scattering the electrons in a predictable manner. Working back from the observed diffraction pattern, it is then possible to deduce the structure of the crystal producing the diffraction pattern. Unlike other types of radiation used in diffraction studies of materials, such as X-rays and neutrons, electrons are charged particles and interact with matter through the Coulomb forces. This means that the incident electrons feel the influence of both the positively charged atomic nuclei and the surrounding electrons. In comparison, X-rays interact with the spatial distribution of the valence electrons, while neutrons are scattered by the atomic nuclei through the strong nuclear force.
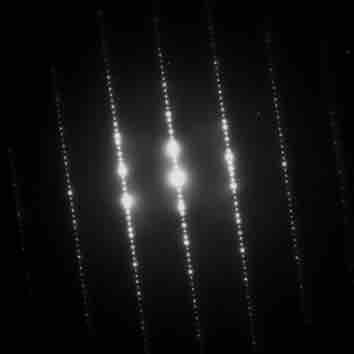
Electron Diffraction Pattern
Typical electron diffraction pattern obtained in a transmission electron microscope with a parallel electron beam.
Neutrons have also been used for studying crystalline structures. They are scattered by the nuclei of the atoms, unlike X-rays, which are scattered by the electrons of the atoms. Thus, neutron diffraction has some key differences compared to more common methods using X-rays or electrons. For example, the scattering of X-rays is highly dependent on the atomic number of the atoms (i.e., the number of electrons), whereas neutron scattering depends on the properties of the nuclei. In addition, the magnetic moment of the neutron is non-zero, and can thus also be scattered by magnetic fields. This means that neutron scattering is more useful for determining the properties of atomic nuclei, despite the fact that neutrons are significantly harder to create, manipulate, and detect compared to X-rays and electrons.