Euthyroid sick syndrome
Euthyroid sick syndrome | |
---|---|
Other names | Sick euthyroid syndrome (SES); thyroid allostasis in critical illness, tumours, uremia and starvation (TACITUS); nonthyroidal illness syndrome (NTIS); low T3 low T4 syndrome |
Specialty | Endocrinology ![]() |
Euthyroid sick syndrome (ESS) is a state of adaptation or dysregulation of thyrotropic feedback control[1] wherein the levels of T3 and/or T4 are abnormal, but the thyroid gland does not appear to be dysfunctional. This condition may result from allostatic responses of hypothalamus-pituitary-thyroid feedback control, dyshomeostatic disorders, drug interferences, and impaired assay characteristics in critical illness.
The classical phenotype of this condition is often seen in starvation, critical illness, or patients in the intensive care unit. Similar endocrine phenotypes are observed in fetal life and in hibernating mammals.[2] The most common hormone pattern in nonthyroidal illness syndrome is low total and free T3, elevated rT3, and normal T4 and TSH levels, although T4 and TSH suppression may occur in more severe or chronic illness.[3] This classical pattern results from type 1 allostatic load, i.e. a stress response resulting from lacking energy, oxygen, and glutathione.[2]
An alternative phenotype with a largely inverse hormonal pattern is seen in several physiological and pathological conditions, including pregnancy, obesity, endurance training, and psychiatric diseases. It is typically associated with high-T3 syndrome, increased plasma protein binding of thyroid hormones, and an elevated set point of the homeostatic system. It represents a response to type-2 allostatic load.[2]
Classical phenotype (type 1 thyroid allostasis)
Causes
Causes of classical euthyroid sick syndrome include a number of acute and chronic conditions, including pneumonia, fasting, starvation, anorexia nervosa, sepsis, trauma,[4] cardiopulmonary bypass, malignancy, stress, heart failure, hypothermia, myocardial infarction, kidney failure, cirrhosis, diabetic ketoacidosis,[1] surgery, infection, brain injury, shock, cancer,[5] and HIV.[6]
Outside the hospital setting, euthyroid sick syndrome (nonthyroidal illness syndrome - NTIS) has been assumed closely related with a series of chronic diseases, such as inflammatory bowel disease,[7] chronic fatigue syndrome,[8] and autoimmune diseases.[5]
Additionally, an NTIS-like phenotype can be present in major depressive disorder,[5] as well as overexercise.[2]
Pathophysiology
In critical illness, the activity of different deiodinases is altered. Humoral and neuronal inputs at the level of the hypothalamus may adjust the set point of thyroid homeostasis. This may play an important role in the pathogenesis of the central component of thyroid allostasis in critical illness, tumors, uremia and starvation (TACITUS).[9] In addition, both illness and medication (e.g. salicylates and heparin) may impair plasma protein binding of thyroid hormones, resulting in reduced levels of total hormones, while free hormone concentrations may be temporarily elevated.
Euthyroid sick syndrome probably represents an overlap of an allostatic response with pathologic reactions and drug interferences.[2] Allostatic overload may result in wasting syndrome and myxedema coma. Thyroid storm, though, represents allostatic failure, where the organism is unable to develop NTIS in the situation of thyrotoxicosis.[2]
Deiodinases
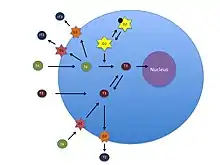
Three primary deiodinases are responsible for thyroid hormone conversion and breakdown. Type 1 (D1) deiodinates T4 to the biologically active T3, as well as the hormonally inactive and possibly inhibitory rT3.[3][5] Type 2 (D2) converts T4 into T3, and breaks down rT3. D3 produces rT3 from T4, and breaks down T3. The balance of D2 and D3 is important for overall T3/rT3 balance.[5][10]
In NTIS, the concentrations of these deiodinases are altered, although whether NTIS is the cause or effect of this in peripheral tissues is unclear; in some studies, the alterations in thyroid hormone concentrations occurred before the changes in deiodinase activity.[5] Typically, peripheral D1 and D2 are downregulated, while peripheral D3 is upregulated; this is associated with lower T4 and increased rT3.[3][5]
Hypothalamic-pituitary-thyroid axis downregulation
Thyrotropin releasing hormone (TRH) neurons in the hypothalamus integrate global signals about the body's energy state. They may be stimulated by signals such as leptin, alpha-MSH, and catecholamines; and inhibited by glucocorticoids, neuropeptide Y, and agouti-related peptide.[2]
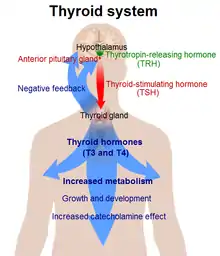
In critical illness, inflammation increases tanycyte D2 in the paraventricular nucleus (PVN) of the hypothalamus, leading to local tissue hyperthyroidism. There may also be decreased central D3.[3][5] This causes negative feedback on the HPT axis, and therefore reduced TRH gene expression in the PVN. This is exemplified by the common NTIS phenotype of low TSH even in the face of peripheral hypothyroidism.[3][5][10][2]
Cytokines
Illness can cause inflammation, which often involves an increase in cytokines such as TNFa, IL-1, and IL-6. Cytokines are implicated in NTIS.[10][3] IL-1β has been shown to decrease liver D1,[10] as well as thyroid hormone receptor (THR) levels. IL-6 and TNFa downregulate D1 and suppress TSH, are negatively correlated with fT3, and are positively correlated with rT3.[3] NF-κB also inhibits D1, and decreases the expression of Thyroid receptors α and β.[3] IFNy inhibits thyroid and Tg release, and also inhibits the upregulation of TSH receptors.[11]
Thyroid hormone receptors
In chronic liver and renal (kidney) failure, increased THR expression occurs. In contrast, in acute illness such as sepsis and trauma, decreased THR expression occurs.[5]
Thyroid hormone transporters
During NTIS, alterations arise in the concentrations of thyroid hormone transporters such as MCT8 and MCT10, although whether the levels are increased or decreased depends on the study. The altered concentrations are thought to be a result of NTIS, rather than a cause; a study in rabbits showed that administering thyroid hormones normalized transporter expression.[5]
Binding proteins
Decreased thyroxine-binding globulin (TBG) occurs following bypass surgery, and in chronic illness, a less effective form of TBG with lower affinity for thyroxine is synthesized. Reduced quantities of bound thyroid result, leading to decreased total thyroid measurements. Decreases in total thyroid may be more severe than alternations in free hormone levels.[5]
Drugs
Dopamine and corticosteroids, commonly given in the hospital setting, can suppress TSH and suppress conversion of T4 to T3.[3][5] Other drugs such as estrogen, contraceptives, salicylates, and phenytoin can alter the binding of TBG to TH, resulting in different TH concentrations.[3] Additionally, lithium disrupts thyroid function,[2] and thyromimetic endocrine disrupters may downregulate the HPT axis.[2]
Fasting
Fasting is a common response in inflammation and critical illness. Originally, selenium deficiency as a result of malnutrition was thought to reduce D1 catalytic activity, but this theory has not been supported as a cause of NTIS.[3][5]
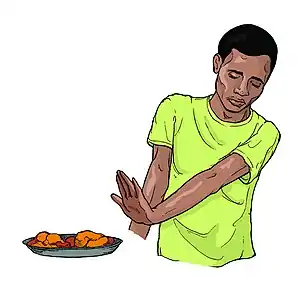
NTIS as a result of fasting may be regarded as a healthy and adaptive mechanism that reduces energy expenditure.[10] Fasting in healthy, euthyroid people causes reduced T3 and elevated rT3, although TSH is usually unchanged.[3][5][10] Even moderate weight loss can lower T3.[2]
This may be primarily via reduced levels of leptin (the satisfaction hormone). Low leptin levels can downregulate hypothalamic TRH neurons and cause a reduction in TSH.[5][10] Ιn fasting animals, administering leptin reverses NTIS symptoms and restores thyroid hormone concentrations.[5] In obesity, increased leptin increases TSH and T3, and lowers rT3, possibly as an attempt to increase energy expenditure and return to weight set point.[2]
Other signals associated with hunger also affect the HPT axis. Insulin and bile acids, which are elevated after a meal, lead to increased D2 activity,[2] therefore increasing T3 and reducing rT3. Low leptin increases NPY and AGRP (associated with appetite), which inhibit TRH gene expression; this effect is enhanced by ghrelin (the hunger hormone).[5] a-MSH stimulates TRH gene expression in the PVN. This is enhanced by leptin, and inhibited by low leptin. a-MSH is also antagonized by AGRP.[5]
Alternative phenotype (type 2 thyroid allostasis)
An anti-NTIS phenotype is observed in some circumstances, wherein TSH, T3, and T4 are generally elevated rather than suppressed. This can occur during pregnancy, obesity, cold adaptation, stay in high altitudes, endurance exercise, acute psychosis, and post-traumatic stress disorder.[2][12]
According to newer theories,[2] elevated concentrations of TSH and thyroid hormones in type 2 allostasis result from an up-regulated set point of the feedback loop, which ensues from increased TRH expression in the basolateral amygdala and the paraventricular nucleus of the hypothalamus in response to stress.[13][14]
High-T3 syndrome in thyroid carcinoma may result from autonomous thyroid hormone secretion or overexpression of type 2 deiodinase in cancer cells rather than from type 2 allostasis.[15][16][17][18]
Conditions with mixed phenotypes
Psychiatry
Stress suppresses TSH,[5] and alterations in thyroid hormone levels may arise in psychiatric illness. In major depressive disorder, an NTIS-like phenotype may be observed, with reduced T3 and increased rT3. T4 may be elevated, and TSH is usually normal, although TSH's normal circadian rhythm may be disrupted.[2] Bipolar 1 and PTSD can exemplify an anti-NTIS phenotype, with upregulation of the HPT axis and increased T3. This may also occur during acute schizophrenic episodes.[2]
Exercise
After exercise, a transient increase occurs in TSH, T4, and T3, but this is thought to be due to increased blood concentration as a result of dehydration.[2] The effects normalize after rest. After long-term heavy strain, levels of thyroid hormones decrease.[2] This is exacerbated by other stressors such as undernutrition and lack of sleep, such as in a military training setting. During endurance exercise, before exhaustion, elevated thyroid hormone levels may happen due to increased expected energy demand (type 2 allostatic load).[2]
Environmental conditions
Cold exposure and stay at high altitude may lead to type 1 or type 2 phenotype, depending on duration and other boundary conditions (which determine whether or not stress is associated with energy deprivation).[12][19]
Diagnosis
Affected patients may have normal, low, or slightly elevated TSH depending on the spectrum and phase of illness. Total T4 and T3 levels may be altered by binding protein abnormalities, and medications. Reverse T3 levels are generally increased, while FT3 is decreased. FT4 levels may have a transient increase, before becoming subnormal during severe illness. Correspondingly, in the majority of cases calculated sum activity of peripheral deiodinases (SPINA-GD) is reduced.[7][20][21][22] Generally the levels of free T3 will be lowered, followed by the lowering of free T4 in more severe disease. Several studies described elevated concentrations of 3,5-T2, an active thyroid hormone, in NTIS.[22][23] 3,5-T2 levels were also observed to correlate with concentrations of rT3 (reverse T3)[22] in patients with euthyroid sick syndrome.
NTIS is a component of a complex endocrine adaptation process, so affected patients might also have hyperprolactinemia and elevated levels of corticosteroids (especially cortisol) and growth hormone. NTIS can be difficult to distinguish from other forms of thyroid dysfunction in the hospital setting. Both NTIS and primary hypothyroidism may have reduced fT3 and fT4, and elevated TSH (which is common in the hospital, during the recovery phase of NTIS).[2] Prescribing thyroxine to treat this may lead to lifelong thyroid overtreatment.[2]
Hyperthyroidism may be assumed due to decreased TSH and a transient fT4 increase. In some cases, this can be distinguished from NTIS by a thyroid ultrasound, which is commonly available in the hospital intensive care unit.[2]
NTIS looks similar to central hypopituitarism; both frequently have reduced TSH and thyroid hormone levels.[2]
Treatment
Debate is ongoing as to whether NTIS is an adaptive or maladaptive mechanism in response to physiological stress.[3][5][10] Some sources indicate that NTIS is beneficial as an acute-phase response, but detrimental during the chronic phase of illness.[2] Several trials have investigated a possible therapy for NTIS, but they yielded inconsistent and partly contradictory results. This may be due to the heterogeneity of investigated populations, and to the lack of a consistent definition of NTIS.[24]
Administering exogenous T3 and T4 has variable results,[3][10] but overall seems to confer no improvements to health outcome.[5] Administering TRH to patients with chronic illness, however, seems to normalize thyroid levels and improve catabolic function.[5]
When NTIS is caused by the normal fasting response to illness, early parenteral nutrition has been shown to attenuate alterations in thyroid hormone (TSH, T3, T4, rT3) levels, whereas late parenteral nutrition exacerbates it.[10] Late parenteral nutrition, though, also reduced complications and accelerated recovery in one study.[10]
History
In 1968, a reduced T4 half-life in athletes was discovered. This was the first awareness of thyroid hormone concentration alterations that were not a result of thyroid gland or pituitary dysfunction. In 1971, they also found a transient increase in T4 during bicycle training.[2]
In 1973, Rothenbuchner et al. discovered that starvation is correlated with reduced T3 concentration. Following this, a similar phenotype was noted in patients with critical illness, tumors, and uremia.[2]
The alternative phenotype of type-2 thyroid allostasis was first predicted in 1968, when John W. Mason expected the concentrations of thyroid hormones to rise in situations of psychosocial stress.[25] Mason's postulate was later confirmed by numerous studies.[26][27][28][29][30][31][32]
See also
References
- 1 2 Hershman JM. "Euthyroid Sick Syndrome: Thyroid Disorders". Merck Manual Professional. Merck & Co., Inc. Retrieved 2009-03-29.
- 1 2 3 4 5 6 7 8 9 10 11 12 13 14 15 16 17 18 19 20 21 22 23 24 25 26 27 Chatzitomaris A, Hoermann R, Midgley JE, Hering S, Urban A, Dietrich B, Abood A, Klein HH, Dietrich JW (20 July 2017). "Thyroid Allostasis-Adaptive Responses of Thyrotropic Feedback Control to Conditions of Strain, Stress, and Developmental Programming". Frontiers in Endocrinology. 8: 163. doi:10.3389/fendo.2017.00163. PMC 5517413. PMID 28775711.
- 1 2 3 4 5 6 7 8 9 10 11 12 13 14 Pappa TA, Vagenakis AG, Alevizaki M (February 2011). "The nonthyroidal illness syndrome in the non-critically ill patient". European Journal of Clinical Investigation. 41 (2): 212–20. doi:10.1111/j.1365-2362.2010.02395.x. PMID 20964678. S2CID 24529523.
- ↑ Dietrich, J. W.; Ackermann, A.; Kasippillai, A.; Kanthasamy, Y.; Tharmalingam, T.; Urban, A.; Vasileva, S.; Schildhauer, T. A.; Klein, H. H.; Stachon, A.; Hering, S. (19 September 2019). "Adaptive Veränderungen des Schilddrüsenstoffwechsels als Risikoindikatoren bei Traumata". Trauma und Berufskrankheit. 21 (4): 260–267. doi:10.1007/s10039-019-00438-z. S2CID 202673793.
- 1 2 3 4 5 6 7 8 9 10 11 12 13 14 15 16 17 18 19 20 21 22 23 Warner MH, Beckett GJ (April 2010). "Mechanisms behind the non-thyroidal illness syndrome: an update". The Journal of Endocrinology. 205 (1): 1–13. doi:10.1677/JOE-09-0412. PMID 20016054.
- ↑ Abelleira E, De Cross GA, Pitoia F (2014). "[Thyroid dysfunction in adults infected by human immunodeficiency virus]". Medicina. 74 (4): 315–20. PMID 25188661.
- 1 2 Liu S, Ren J, Zhao Y, Han G, Hong Z, Yan D, Chen J, Gu G, Wang G, Wang X, Fan C, Li J (February 2013). "Nonthyroidal illness syndrome: is it far away from Crohn's disease?". Journal of Clinical Gastroenterology. 47 (2): 153–9. doi:10.1097/MCG.0b013e318254ea8a. PMID 22874844. S2CID 35344744.
- ↑ Ruiz-Núñez B, Tarasse R, Vogelaar EF, Janneke Dijck-Brouwer DA, Muskiet FA (20 March 2018). "Higher Prevalence of "Low T3 Syndrome" in Patients With Chronic Fatigue Syndrome: A Case-Control Study". Frontiers in Endocrinology. 9: 97. doi:10.3389/fendo.2018.00097. PMC 5869352. PMID 29615976.
- ↑ Hoermann R, Midgley JE, Larisch R, Dietrich JW (2015). "Homeostatic Control of the Thyroid-Pituitary Axis: Perspectives for Diagnosis and Treatment". Frontiers in Endocrinology. 6: 177. doi:10.3389/fendo.2015.00177. PMC 4653296. PMID 26635726.
- 1 2 3 4 5 6 7 8 9 10 11 Fliers E, Bianco AC, Langouche L, Boelen A (October 2015). "Thyroid function in critically ill patients". The Lancet. Diabetes & Endocrinology. 3 (10): 816–25. doi:10.1016/S2213-8587(15)00225-9. PMC 4979220. PMID 26071885.
- ↑ de Vries EM, Fliers E, Boelen A (June 2015). "The molecular basis of the non-thyroidal illness syndrome". The Journal of Endocrinology. 225 (3): R67–81. doi:10.1530/JOE-15-0133. PMID 25972358.
- 1 2 Alhazmi, Ayman S; Alomey, Anas; Almalki, Hilal; Almehmadi, Mazen; Albshri, Mohammad; Algarni, Naif; Almutiri, Sultan (December 2018). "Effect of High Altitude on Thyroid and Thyroid Stimulating Hormones Levels in Taif City" (PDF). Acta Scientific Nutritional Health. Acta Scientific. 2 (12). Retrieved 22 September 2019.
- ↑ Choi, Juli; Kim, Ji-eun; Kim, Tae-Kyung; Park, Jin-Young; Lee, Jung-Eun; Kim, Hannah; Lee, Eun-Hwa; Han, Pyung-Lim (October 2015). "TRH and TRH receptor system in the basolateral amygdala mediate stress-induced depression-like behaviors". Neuropharmacology. 97: 346–356. doi:10.1016/j.neuropharm.2015.03.030. PMID 26107116. S2CID 1839062.
- ↑ Wittmann, Gábor; Lechan, Ronald M.; Liposits, Zsolt; Fekete, Csaba (March 2005). "Glutamatergic innervation of corticotropin-releasing hormone- and thyrotropin-releasing hormone-synthesizing neurons in the hypothalamic paraventricular nucleus of the rat". Brain Research. 1039 (1–2): 53–62. doi:10.1016/j.brainres.2005.01.090. PMID 15781046. S2CID 14375998.
- ↑ Kim, BW; Daniels, GH; Harrison, BJ; Price, A; Harney, JW; Larsen, PR; Weetman, AP (February 2003). "Overexpression of type 2 iodothyronine deiodinase in follicular carcinoma as a cause of low circulating free thyroxine levels". The Journal of Clinical Endocrinology and Metabolism. 88 (2): 594–8. doi:10.1210/jc.2002-020921. PMID 12574186.
- ↑ Miyauchi, A; Takamura, Y; Ito, Y; Miya, A; Kobayashi, K; Matsuzuka, F; Amino, N; Toyoda, N; Nomura, E; Nishikawa, M (June 2008). "3,5,3'-Triiodothyronine thyrotoxicosis due to increased conversion of administered levothyroxine in patients with massive metastatic follicular thyroid carcinoma". The Journal of Clinical Endocrinology and Metabolism. 93 (6): 2239–42. doi:10.1210/jc.2007-2282. PMID 18397985.
- ↑ Abid, SA; Stack BC, Jr; Bodenner, DL (2014). "Metastatic Follicular Thyroid Carcinoma Secreting Thyroid Hormone and Radioiodine Avid without Stimulation: A Case Report and Literature Review". Case Reports in Endocrinology. 2014: 584513. doi:10.1155/2014/584513. PMC 4220571. PMID 25400957.
- ↑ Yu, Run (2018). "High T3 Syndrome Associated with Metastatic Papillary and Poorly Differentiated Thyroid Cancer" (PDF). Archives of Diabetes and Endocrine System. 1 (1): 10–12. doi:10.22259/2638-4981.0101003. Retrieved 22 September 2019.
- ↑ Hackney, AC; Feith, S; Pozos, R; Seale, J (April 1995). "Effects of high altitude and cold exposure on resting thyroid hormone concentrations". Aviation, Space, and Environmental Medicine. 66 (4): 325–9. PMID 7794224.
- ↑ Rosolowska-Huszcz D, Kozlowska L, Rydzewski A (August 2005). "Influence of low protein diet on nonthyroidal illness syndrome in chronic renal failure". Endocrine. 27 (3): 283–8. doi:10.1385/ENDO:27:3:283. PMID 16230785. S2CID 25630198.
- ↑ Han G, Ren J, Liu S, Gu G, Ren H, Yan D, Chen J, Wang G, Zhou B, Wu X, Yuan Y, Li J (September 2013). "Nonthyroidal illness syndrome in enterocutaneous fistulas". American Journal of Surgery. 206 (3): 386–92. doi:10.1016/j.amjsurg.2012.12.011. PMID 23809674.
- 1 2 3 Dietrich JW, Müller P, Schiedat F, Schlömicher M, Strauch J, Chatzitomaris A, Klein HH, Mügge A, Köhrle J, Rijntjes E, Lehmphul I (June 2015). "Nonthyroidal Illness Syndrome in Cardiac Illness Involves Elevated Concentrations of 3,5-Diiodothyronine and Correlates with Atrial Remodeling". European Thyroid Journal. 4 (2): 129–37. doi:10.1159/000381543. PMC 4521060. PMID 26279999.
- ↑ Pinna G, Meinhold H, Hiedra L, Thoma R, Hoell T, Gräf KJ, Stoltenburg-Didinger G, Eravci M, Prengel H, Brödel O, Finke R, Baumgartner A (May 1997). "Elevated 3,5-diiodothyronine concentrations in the sera of patients with nonthyroidal illnesses and brain tumors". The Journal of Clinical Endocrinology and Metabolism. 82 (5): 1535–42. doi:10.1210/jcem.82.5.3939. PMID 9141546.
- ↑ Dietrich JW, Stachon A, Antic B, Klein HH, Hering S (October 2008). "The AQUA-FONTIS study: protocol of a multidisciplinary, cross-sectional and prospective longitudinal study for developing standardized diagnostics and classification of non-thyroidal illness syndrome". BMC Endocrine Disorders. 8: 13. doi:10.1186/1472-6823-8-13. PMC 2576461. PMID 18851740.
- ↑ MASON, JOHN W. (September 1968). "A Review of Psychoendocrine Research on the Pituitary-Adrenal Cortical System". Psychosomatic Medicine. 30 (5): 576–607. doi:10.1097/00006842-196809000-00020. PMID 4303377. S2CID 26755389.
- ↑ Levy, RP; Jensen, JB; Laus, VG; Agle, DP; Engel, IM (November 1981). "Serum thyroid hormone abnormalities in psychiatric disease". Metabolism: Clinical and Experimental. 30 (11): 1060–4. doi:10.1016/0026-0495(81)90048-2. PMID 7289880.
- ↑ Spratt, DI; Pont, A; Miller, MB; McDougall, IR; Bayer, MF; McLaughlin, WT (July 1982). "Hyperthyroxinemia in patients with acute psychiatric disorders". The American Journal of Medicine. 73 (1): 41–8. doi:10.1016/0002-9343(82)90922-6. PMID 6807087.
- ↑ Chopra, IJ; Solomon, DH; Huang, TS (May 1990). "Serum thyrotropin in hospitalized psychiatric patients: evidence for hyperthyrotropinemia as measured by an ultrasensitive thyrotropin assay". Metabolism: Clinical and Experimental. 39 (5): 538–43. doi:10.1016/0026-0495(90)90014-4. PMID 2336036.
- ↑ Wang, S; Mason, J; Southwick, S; Johnson, D; Lubin, H; Charney, D (July–August 1995). "Relationships between thyroid hormones and symptoms in combat-related posttraumatic stress disorder". Psychosomatic Medicine. 57 (4): 398–402. doi:10.1097/00006842-199507000-00012. PMID 7480570. S2CID 20905167.
- ↑ Friedman, MJ; Wang, S; Jalowiec, JE; McHugo, GJ; McDonagh-Coyle, A (15 May 2005). "Thyroid hormone alterations among women with posttraumatic stress disorder due to childhood sexual abuse". Biological Psychiatry. 57 (10): 1186–92. doi:10.1016/j.biopsych.2005.01.019. PMID 15866559. S2CID 23455036.
- ↑ Mason, J; Southwick, S; Yehuda, R; Wang, S; Riney, S; Bremner, D; Johnson, D; Lubin, H; Blake, D; Zhou, G (August 1994). "Elevation of serum free triiodothyronine, total triiodothyronine, thyroxine-binding globulin, and total thyroxine levels in combat-related posttraumatic stress disorder". Archives of General Psychiatry. 51 (8): 629–41. doi:10.1001/archpsyc.1994.03950080041006. PMID 8042912.
- ↑ Karlović, D; Marusić, S; Martinac, M (30 June 2004). "Increase of serum triiodothyronine concentration in soldiers with combat-related chronic post-traumatic stress disorder with or without alcohol dependence". Wiener klinische Wochenschrift. 116 (11–12): 385–90. doi:10.1007/BF03040918. PMID 15291291. S2CID 26011489.
Further reading
- McIver B, Gorman CA (February 1997). "Euthyroid sick syndrome: an overview". Thyroid. 7 (1): 125–32. doi:10.1089/thy.1997.7.125. PMID 9086580.