Rhodococcus
Rhodococcus | |
---|---|
![]() | |
Rhodococcus sp. | |
Scientific classification | |
Domain: | |
Phylum: | |
Class: | |
Order: | |
Family: | |
Genus: | Rhodococcus Zopf 1891 |
Rhodococcus is a genus of aerobic, nonsporulating, nonmotile Gram-positive bacteria closely related to Mycobacterium and Corynebacterium.[1][2] While a few species are pathogenic, most are benign, and have been found to thrive in a broad range of environments, including soil, water, and eukaryotic cells. Some species have large genomes, including the 9.7 megabasepair genome (67% G/C) of Rhodococcus sp. RHA1.[3]
Strains of Rhodococcus are important owing to their ability to catabolize a wide range of compounds and produce bioactive steroids, acrylamide, and acrylic acid, and their involvement in fossil fuel biodesulfurization.[3] This genetic and catabolic diversity is not only due to the large bacterial chromosome, but also to the presence of three large linear plasmids.[1] Rhodococcus is also an experimentally advantageous system owing to a relatively fast growth rate and simple developmental cycle, but is not well characterized.[3]
Another important application of Rhodococcus comes from bioconversion, using biological systems to convert cheap starting material into more valuable compounds, such as its ability to metabolize harmful environmental pollutants, including toluene, naphthalene, herbicides, and PCBs. Rhodococcus species typically metabolize aromatic substrates by first oxygenating the aromatic ring to form a diol (two alcohol groups). Then, the ring is cleaved with intra/extradiol mechanisms, opening the ring and exposing the substrate to further metabolism. Since the chemistry is very stereospecific, the diols are created with predictable chirality. While controlling the chirality of chemical reaction presents a significant challenge for synthetic chemists, biological processes can be used instead to faithfully produce chiral molecules in cases where direct chemical synthesis is not feasible or efficient. An example of this is the use of Rhodococcus to produce indene, a precursor to the AIDS drug indinavir, a protease inhibitor, and containing two of the five chiral centers needed in the complex.[4]

Biodegradation of organic pollutants
Rhodococcus has been greatly researched as a potential agent for the bioremediation of pollutants as it is commonly found in the natural environment, and they possess certain characteristics that allow them to thrive under a variety of conditions, and they have the capability to metabolize many hydrocarbons.[5]
Rhodococci possess many properties that makes them suitable for bioremediation under a range of environments. Their ability to undergo microaerophilic respiration allows them to survive in environments containing low oxygen concentrations, and their ability to undergo aerobic respiration also allows them to survive in oxygenated environments.[6] They also undergo nitrogen fixation, which allows them to generate their own nutrients in environments with low nutrients.[7]
Rhodococci also contain characteristics that enhances their ability to degrade organic pollutants. Their hydrophobic surface allows for adhesion to hydrocarbons, which enhances its ability to degrade these pollutants.[8] They have a wide variety of catabolic pathways and many unique enzyme functions.[9] This gives them the ability to degrade many recalcitrant, toxic hydrocarbons. For example, Rhodococci expresses dioxygenases, which can be used to degrade benzotrifluoride, a recalcitrant pollutant.[10] Rhodococcus sp. strain Q1, a strain naturally found in soil and paper mill sludge, contains the ability to degrade quinoline, various pyridine derivatives, catechol, benzoate, and protocatechuic acid.[11] Rhodococci are also capable of accumulating heavy metal ions, such as radioactive caesium, allowing for easier removal from the environment.[12] Other pollutants, such as azo dyes,[13] pesticides[14] and polychlorinated biphenyls[15] can also be degraded by Rhodococci.
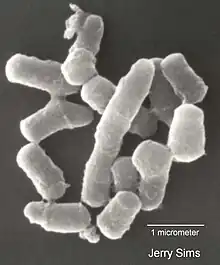
Pathogenic Rhodococcus
The genus Rhodococcus has two pathogenic species: R. fascians and R. equi. The former, a plant pathogen, causes leafy gall disease in both angiosperm and gymnosperm plants.[16] R. equi is the causative agent of foal pneumonia (rattles) and mainly infects foals up to three months in age. However, it has a wide host range, sporadically infecting pigs, cattle, and immunocompromised humans, in particular AIDS patients and those undergoing immunosuppressive therapy.[17] Both pathogens rely on a conjugative virulence plasmid to cause disease. In case of R. fascians, this is a linear plasmid, whereas R. equi harbors a circular plasmid. Both pathogens are economically significant. R. fascians is a major pathogen of tobacco plants. R. equi, one of the most important foal pathogens, is endemic on many stud farms around the world.
In molecular biology
Rhodococcus has also been identified as a contaminant of DNA extraction kit reagents and ultrapure water systems, which may lead to its erroneous appearance in microbiota or metagenomic datasets.[18]
Species
- Rhodococcus aerolatus Hwang et al. 2015[19]
- Rhodococcus aetherivorans Goodfellow et al. 2004[19]
- Rhodococcus agglutinans Guo et al. 2015[19]
- Rhodococcus aurantiacus (ex Tsukamura and Mizuno, 1971) Tsukamura and Yano, 1985, nom. rev.
- Rhodococcus artemisiae Zhao et al. 2012[19]
- Rhodococcus baikonurensis Li, et al., 2004
- Rhodococcus biphenylivorans Su et al. 2015[19]
- Rhodococcus boritolerans
- Rhodococcus equi (Magnusson, 1923) Goodfellow and Alderson, 1977
- Rhodococcus canchipurensis Nimaichand et al. 2013[19]
- Rhodococcus cerastii Kämpfer et al. 2013[19]
- Rhodococcus cercidiphylli Li et al. 2012[19]
- Rhodococcus coprophilus Rowbotham and Cross, 1979
- Rhodococcus corynebacterioides (Serrano, et al., 1972) Yassin and Schaal, 2005 (synonym: Nocardia corynebacterioides (Serrano et al. 1972)
- Rhodococcus defluvii Kämpfer et al. 2014[19]
- Rhodococcus electrodiphilus Ramaprasad et al., 2018[20]
- Rhodococcus enclensis Dastager et al., 2014[19]
- Rhodococcus erythropolis (Gray and Thornton, 1928) Goodfellow and Alderson, 1979
- Rhodococcus fascians (Tilford 1936) Goodfellow 1984 (synonym: Rhodococcus luteus (ex Söhngen 1913) Nesterenko et al. 1982)[21]
- Rhodococcus globerulus Goodfellow, et al., 1985
- Rhodococcus gordoniae Jones, et al., 2004
- Rhodococcus hoagii Kämpfer et al. 2014[19]
- Rhodococcus imtechensis Ghosh et al. 2006[19]
- Rhodococcus jialingiae Wang et al. 2010[19]
- Rhodococcus jostii Takeuchi, et al., 2002. Identified as producing a lignin digesting enzyme, it was the first isolated from a bacterium rather than a fungus.[22][23]
- Rhodococcus koreensis Yoon, et al., 2000
- Rhodococcus kroppenstedtii Mayilraj, et al., 2006
- Rhodococcus kunmingensis Wang et al., 2008[19]
- Rhodococcus kyotonensis Li et al., 2007[19]
- Rhodococcus maanshanensis Zhang, et al., 2002
- Rhodococcus marinonascens Helmke and Weyland, 1984
- Rhodococcus nanhaiensis
- Rhodococcus olei Chaudhary and Kim, 2018[24]
- Rhodococcus opacus Klatte, et al., 1995
- Rhodococcus percolatus Briglia, et al., 1996
- Rhodococcus phenolicus Rehfuss and Urban, 2006
- Rhodococcus polyvorum Li et al. 2012[19]
- Rhodococcus pyridinivorans Yoon, et al., 2000
- Rhodococcus qingshengii Xu et al. 2007[19]
- Rhodococcus rhodochrous (Zopf 1891) Tsukamura, 1974
- Rhodococcus rhodnii Goodfellow and Alderson, 1979 (synonym: Nocardia rhodnii)
- Rhodococcus ruber (Kruse 1896) Goodfellow and Alderson, 1977 (synonym: Streptothrix rubra Kruse, 1896)
- Rhodococcus jostii RHA1
- Rhodococcus soli Li et al. 2015[19]
- Rhodococcus triatomae Yassin, 2005
- Rhodococcus trifolii Kämpfer et al. 2013[19]
- Rhodococcus tukisamuensis Matsuyama, et al., 2003
- Rhodococcus wratislaviensis (Goodfellow et al. 1995) Goodfellow, et al., 2002 (synonym: Tsukamurella wratislaviensis Goodfellow, et al., 1995)
- Rhodococcus yunnanensis Zhang, et al., 2005
- Rhodococcus zopfii Stoecker, et al., 1994
References
- 1 2 van der Geize R. & L. Dijkhuizen (2004). "Harnessing the catabolic diversity of rhodococci for environmental and biotechnological applications". Microbiology. 7 (3): 255–261. doi:10.1016/j.mib.2004.04.001. hdl:11370/a1dfa0fd-dd65-4c1d-b9b4-bfa98038dcbe. PMID 15196492.
- ↑ Burkovski A (editor). (2008). Corynebacteria: Genomics and Molecular Biology. Caister Academic Press. ISBN 978-1-904455-30-1. .
{{cite book}}
:|author=
has generic name (help) - 1 2 3 McLeod MP, Warren RL, Hsiao WW, Araki N, Mihre M, Fernandes C, Miyazawa D, Wong W, Lillquist AL, Wang D, Dosanjh M, Hara H, Petrescu A, Morin RD, Yang G, Stott JM, Schein JE, Shin H, Smailus D, Siddiqui AS, Marra MA, Jones SJ, Holt R, Brinkman FS, Miyauchi K, Fukuda M, Davies JE, Mohn WW, Eltis LD (October 17, 2006). "The complete genome of Rhodococcus sp. RHA1 provides insights into a catabolic powerhouse". PNAS. 103 (42): 15582–15587. Bibcode:2006PNAS..10315582M. doi:10.1073/pnas.0607048103. PMC 1622865. PMID 17030794.
- 1 2 Treadway, S.L., K.S. Yanagimachi, E. Lankenau, P.A. Lessard, G. Stephanopoulos and A.J. Sinskey (1999). "Isolation and characterization of indene bioconversion genes from Rhodococcus strain I24". Appl. Microbiol. Biotechnol. 51 (6): 786–793. doi:10.1007/s002530051463. PMID 10422226. S2CID 6264248.
{{cite journal}}
: CS1 maint: multiple names: authors list (link) - ↑ Alvarez, Héctor (2010). Biology of Rhodococcus. Springer Science & Business Media. pp. 231–256. ISBN 9783642129377.
- ↑ Fuller, M.E.; Perreault, N. (July 8, 2010). "Microaerophilic degradation of hexahydro-1,3,5-trinitro-1,3,5-triazine (RDX) by three Rhodococcus strains". Letters in Applied Microbiology. 51 (3): 313–318. doi:10.1111/j.1472-765x.2010.02897.x. PMID 20666987.
- ↑ Blasco, Rafael (2001). "Rhodococcus sp. RB1 grows in the presence of high nitrate and nitrite concentrations and assimilates nitrate in moderately saline environments". Archives of Microbiology. 175 (6): 435–440. doi:10.1007/s002030100285. PMID 11491084. S2CID 864067.
- ↑ Mendez-Volas, A. (2012). Microbes in applied research; current advances and challenges; proceedings. World Scientific. pp. 197–200. ISBN 9789814405034.
- ↑ Laczi, Krisztián; Kis, Ágnes; Horváth, Balázs; Maróti, Gergely; Hegedüs, Botond (November 2015). "Metabolic responses of Rhodococcus erythropolis PR4 grown on diesel oil and various hydrocarbons" (PDF). Applied Microbiology and Biotechnology. 99 (22): 9745–9759. doi:10.1007/s00253-015-6936-z. PMID 26346267. S2CID 9213608.
- ↑ Yano, Kenichi; Wachi, Masaaki; Tsuchida, Sakiko; Kitazume, Tomoya; Iwai, Noritaka (2015). "Degradation of benzotrifluoride via the dioxygenase pathway in Rhodococcus sp. 065240". Bioscience, Biotechnology, and Biochemistry. 79 (3): 496–504. doi:10.1080/09168451.2014.982502. ISSN 1347-6947. PMID 25412819. S2CID 205616972.
- ↑ O'Loughlin, E.J.; Kehrmeyer, S.R.; Sims, G.K. (1996). "Isolation, characterization, and substrate utilization of a quinoline degrading bacterium". International Biodeterioration and Biodegradation. 38 (2): 107–118. doi:10.1016/S0964-8305(96)00032-7.
- ↑ Takei, Takayuki; Yamasaki, Mika; Yoshida, Masahiro (2014-04-01). "Cesium accumulation of Rhodococcus erythropolis CS98 strain immobilized in hydrogel matrices". Journal of Bioscience and Bioengineering. 117 (4): 497–500. doi:10.1016/j.jbiosc.2013.09.013. PMID 24183457.
- ↑ Heiss, G. S.; Gowan, B.; Dabbs, E. R. (1992-12-01). "Cloning of DNA from a Rhodococcus strain conferring the ability to decolorize sulfonated azo dyes". FEMS Microbiology Letters. 78 (2–3): 221–226. doi:10.1016/0378-1097(92)90030-r. ISSN 0378-1097. PMID 1490602.
- ↑ Parekh, N. R.; Walker, A.; Roberts, S. J.; Welch, S. J. (November 1994). "Rapid degradation of the triazinone herbicide metamitron by a Rhodococcus sp. isolated from treated soil". The Journal of Applied Bacteriology. 77 (5): 467–475. doi:10.1111/j.1365-2672.1994.tb04389.x. ISSN 0021-8847. PMID 8002472.
- ↑ Boyle, Alfred W.; Silvin, Christopher J.; Hassett, John P.; Nakas, James P.; Tanenbaum, S. W. (1992-06-01). "Bacterial PCB biodegradation". Biodegradation. 3 (2–3): 285–298. doi:10.1007/BF00129089. ISSN 0923-9820. S2CID 7272347.
- ↑ Goethals, K.; Vereecke, D.; Jaziri, M.; Van, Montagu M.; Holsters, M. (2001). "Leafy gall formation by Rhodococcus fascians". Annu. Rev. Phytopathol. 39: 27–52. doi:10.1146/annurev.phyto.39.1.27. PMID 11701858.
- ↑ Muscatello, G.; Leadon, D. P.; Klay, M.; Ocampo-Sosa, A.; Lewis, D. A.; Fogarty, U.; Buckley, T.; Gilkerson, J. R.; Meijer, W. G.; et al. (2007). "Rhodococcus equi infection in foals: the science of 'rattles'". Equine Vet. J. 39 (5): 470–478. doi:10.2746/042516407x209217. PMID 17910275.
- ↑ Salter, S; Cox, M; Turek, E; Calus, S; Cookson, W; Moffatt, M; Turner, P; Parkhill, J; Loman, N; Walker, A (2014). "Reagent contamination can critically impact sequence-based microbiome analyses". bioRxiv 10.1101/007187.
- 1 2 3 4 5 6 7 8 9 10 11 12 13 14 15 16 17 18 19 Parte, A.C. "Rhodococcus". LPSN.
- ↑ Ramaprasad, E. V. V.; Mahidhara, Ganesh; Sasikala, Ch.; Ramana, Ch. V. (2018). "Rhodococcus electrodiphilus sp. nov., a marine electro active actinobacterium isolated from coral reef". International Journal of Systematic and Evolutionary Microbiology. 68 (8): 2644–2649. doi:10.1099/ijsem.0.002895. PMID 29957174.
- ↑ Klatte, S.; et al. (1994). "Rhodococcus luteus is a later subjective synonym of Rhodococcus fascians". Int. J. Syst. Bacteriol. 44 (4): 627–630. doi:10.1099/00207713-44-4-627.
- ↑ "First wood-digesting enzyme found in bacteria could boost biofuel production".
- ↑ Takeuchi, M; Hatano, K; Sedlácek, I; Pácová, Z (2002). "Rhodococcus jostii sp. nov., isolated from a medieval grave". International Journal of Systematic and Evolutionary Microbiology. 52 (Pt 2): 409–13. doi:10.1099/00207713-52-2-409. PMID 11931149.
- ↑ Chaudhary, Dhiraj Kumar; Kim, Jaisoo (2018). "Rhodococcus olei sp. nov., with the ability to degrade petroleum oil, isolated from oil-contaminated soil". International Journal of Systematic and Evolutionary Microbiology. 68 (5): 1749–1756. doi:10.1099/ijsem.0.002750. PMID 29620494.
External links
- For species and synonyms see here: National Center for Biotechnology Information (NCBI)