Linear acetylenic carbon
Linear acetylenic carbon (LAC), also known as carbyne or Linear Carbon Chain (LCC), is an allotrope of carbon that has the chemical structure (−C≡C−)n as a repeat unit, with alternating single and triple bonds.[1][2] It would thus be the ultimate member of the polyyne family.
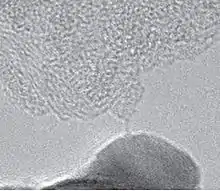
This polymeric carbyne is of considerable interest to nanotechnology as its Young's modulus is 32.7 TPa – forty times that of diamond;[4] this extraordinary number is, however, based on a novel definition of cross-sectional area that does not correspond to the space occupied by the structure. Carbyne has also been identified in interstellar space; however, its existence in condensed phases has been contested recently, as such chains would crosslink exothermically (and perhaps explosively) if they approached each other.[5]
History and controversy
The first claims of detection of this allotrope were made in 1960[5][6] and repeated in 1978.[7] A 1982 re-examination of samples from several previous reports determined that the signals originally attributed to carbyne were in fact due to silicate impurities in the samples.[8] Absence of carbyne crystalline rendered the direct observation of a pure carbyne-assembled solid still a major challenge, because carbyne crystals with well-defined structures and sufficient sizes are not available to date. This is indeed the major obstacle to general acceptance of carbyne as a true carbon allotrope. The mysterious carbyne still attracted scientists with its possible extraordinary properties.[9]
During the past thirty five years an increasing body of experimental and theoretical work has been published in the scientific literature dealing with the preparation of carbyne and the study of its structure, properties and potential applications.[10][11] In 1968 a silver-white new mineral was discovered in graphitic gneisses of the Ries Crater (Nordlingen, Bavaria, Germany).[12] This material was found to consist entirely of carbon and its hexagonal cell dimensions matched those reported earlier for carbine by Russians scientists.[13] It was concluded that this novel form of natural carbon, chaoite, was generated from graphite by the combined action of high temperature and high pressure, presumably caused by the impact of meteorite. Soon afterwards this “white” carbon was synthesized by sublimation of pyrolytic graphite in vacuum.[14]
In 1984, a group at Exxon reported the detection of clusters with even numbers of carbons, between 30 and 180, in carbon evaporation experiments, and attributed them to polyyne carbon.[15] However, these clusters later were identified as fullerenes.[5]
In 1991, carbyne was allegedly detected among various other allotropes of carbon in samples of amorphous carbon black vaporized and quenched by shock waves produced by shaped explosive charges.[16]
In 1995, the preparation of carbyne chains with over 300 carbons was reported. They were claimed to be reasonably stable, even against moisture and oxygen, as long as the terminal alkynes on the chain are capped with inert groups (such as tert-butyl or trifluoromethyl) rather than hydrogen atoms. The study claimed that the data specifically indicated a carbyne-like structures rather than fullerene-like ones.[17] However, according to H. Kroto, the properties and synthetic methods used in those studies are consistent with generation of fullerenes.[5]
Another 1995 report claimed detection of carbyne chains of indeterminate length in a layer of carbonized material, about 180 nm thick, resulting from the reaction of solid polytetrafluoroethylene (PTFE, Teflon) immersed in alkali metal amalgam at ambient temperature (with no hydrogen-bearing species present).[18] The assumed reaction was
- (-CF2−CF2-)n + 4M → (-C≡C-)n + 4MF,
where M is either lithium, sodium, or potassium. The authors conjectured that nanocrystals of the metal fluoride between the chains prevented their polymerization.
In 1999, it was reported that copper(I) acetylide (Cu+2 C2−2), after partial oxidation by exposure to air or copper(II) ions followed by decomposition with hydrochloric acid, leaves a "carbonaceous" residue with the spectral signature of (−C≡C−)n chains with n=2–6. The proposed mechanism involves oxidative polymerization of the acetylide anions C2−2 into carbyne-type anions C(≡C−C≡)nC2− or cumulene-type anions C(=C=C=)mC4−.[19] Also, thermal decomposition of copper acetylide in vacuum yielded a fluffy deposit of fine carbon powder on the walls of the flask, which, on the basis of spectral data, was claimed to be carbyne rather than graphite.[19] Finally, the oxidation of copper acetylide in ammoniacal solution (Glaser's reaction) produces a carbonaceous residue that was claimed to consist of "polyacetylide" anions capped with residual copper(I) ions,
- Cu+ C−(≡C−C≡)nC− Cu+.
On the basis of the residual amount of copper, the mean number of units n was estimated to be around 230.[20]
In 2004, an analysis of a synthesized linear carbon allotrope found it to have a cumulene electronic structure—sequential double bonds along an sp-hybridized carbon chain—rather than the alternating triple–single pattern of linear carbyne.[21]
In 2016, the synthesis of linear chains of up to 6,000 sp-hybridized carbon atoms was reported. The chains were grown inside double-walled carbon nanotubes, and are highly stable protected by their hosts.[22][23]
Polyynes
While the existence of "carbyne" chains in pure neutral carbon material is still disputed, short (−C≡C−)n chains are well established as substructures of larger molecules (polyynes).[24] As of 2010, the longest such chain in a stable molecule had 22 acetylenic units (44 atoms), stabilized by rather bulky end groups.[25]
Structure
The carbon atoms in this form are each linear in geometry with sp orbital hybridisation. The estimated length of the bonds is 120.7 pm (triple) and 137.9 pm (single).[18]
Other possible configurations for a chain of carbon atoms include polycumulene (polyethylene-diylidene) chains with double bonds only (128.2 pm). This chain is expected to have slightly higher energy, with a Peierls gap of 2–5 eV. For short Cn molecules, however, the polycumulene structure seems favored. When n is even, two ground configurations, very close in energy, may coexist: one linear, and one cyclic (rhombic).[18]
The limits of flexibility of the carbyne chain are illustrated by a synthetic polyyne with a backbone of 8 acetylenic units, whose chain was found to be bent by 25° or more (about 3° at each carbon) in the solid state, to accommodate the bulky end groups of adjacent molecules.[26]
The highly symmetric carbyne chain is expected to have only one Raman-active mode with Σg symmetry, due to stretching of bonds in each single-double pair, with frequency typically between 1800 and 2300 cm−1,[18] and affected by their environments.[27]
Properties
Carbyne chains have been claimed to be the strongest material known per density. Calculations indicate that carbyne's specific tensile strength (strength divided by density) of (6.0–7.5)×107 (N⋅m)/kg beats graphene ((4.7–5.5)×107 (N⋅m)/kg), carbon nanotubes ((4.3–5.0)×107 (N⋅m)/kg), and diamond ((2.5–6.5)×107 (N⋅m)/kg).[28][29][30] Its specific modulus (Young's Modulus divided by density) of around 109 (N⋅m)/kg is also double that of graphene, which is around 4.5×108 (N⋅m)/kg.[28][30]
Stretching carbyne 10% alters its electronic band gap from 3.2–4.4 eV.[31] Outfitted with molecular handles at chain's ends, it can also be twisted to alter its band gap. With a 90° end-to-end twist, carbyne turns into a magnetic semiconductor.[29]
In 2017, the band gaps of confined linear carbon chains (LCC) inside double-walled carbon nanotubes with lengths ranging from 36 up to 6000 carbon atoms were determined for the first time ranging from 2.253–1.848 eV, following a linear relation with Raman frequency. This lower bound is the smallest band gap of linear carbon chains observed so far. In 2020, the strength (Young's modulus) of linear carbon chains (LCC) was experimentally calculated to be about 20 TPa which is much higher than that of other carbon materials like graphene and carbon nanotubes.[32] The comparison with experimental data obtained for short chains in gas phase or in solution demonstrates the effect of the DWCNT encapsulation, leading to an essential downshift of the band gap.[33]
The LCCs inside double-walled carbon nanotubes lead to an increase of the photoluminescence (PL) signal of the inner tubes up to a factor of 6 for tubes with (8,3) chirality. This behavior can be attributed to a local charge transfer from the inner tubes to the carbon chains, counterbalancing quenching mechanisms induced by the outer tubes.[34]
Carbyne chains can take on side molecules that may make the chains suitable for energy[29] and hydrogen[35] storage.
With a differential Raman scattering cross section of 10−22 cm2 sr−1 per atom, carbyne chains confined inside carbon nanotubes are the strongest Raman scatterer ever reported,[36] exceeding any other know material by two orders of magnitude.
References
- Kudryavtsev, Yu P. (1999). "The Discovery of Carbyne". In Heimann, Robert B.; Evsyukov, Sergey E.; Kavan, Ladislav (eds.). Carbyne and Carbynoid Structures. Physics and Chemistry of Materials with Low-Dimensional Structures. Vol. 21. Dordrecht, The Netherlands: Springer. pp. 1–6. doi:10.1007/978-94-011-4742-2_1. ISBN 0-7923-5323-4.
- Baughman, R. H. (2006). "CHEMISTRY: Dangerously Seeking Linear Carbon". Science. 312 (5776): 1009–1110. doi:10.1126/science.1125999. PMID 16709775. S2CID 93868586.
- La Torre, A.; Botello-Mendez, A.; Baaziz, W.; Charlier, J. -C.; Banhart, F. (2015). "Strain-induced metal–semiconductor transition observed in atomic carbon chains". Nature Communications. 6: 6636. Bibcode:2015NatCo...6.6636L. doi:10.1038/ncomms7636. PMC 4389248. PMID 25818506.
- Itzhaki, L.; Altus, E.; Basch, H.; Hoz, S. (2005). "Harder than Diamond: Determining the Cross-Sectional Area and Young's Modulus of Molecular Rods". Angewandte Chemie. 117 (45): 7598. Bibcode:2005AngCh.117.7598I. doi:10.1002/ange.200502448. Itzhaki, L.; Altus, E.; Basch, H.; Hoz, S. (2005). "Harder than Diamond: Determining the Cross-Sectional Area and Young's Modulus of Molecular Rods". Angewandte Chemie International Edition. 44 (45): 7432–7435. doi:10.1002/anie.200502448. PMID 16240306.
- Kasatockin V.I., Koudryavtsev Y.P, Sladkov A.M, Korshak V.V Inventor's sertification, N°107 (07/12/1971), priority date 06/11/1960
- Sladkov A.M, Kudryavtsev Y.P Diamond, graphite, carbyne 3/4 the allotropic forms of carbon, [J], Priroda (Nature), 1969, 58:37-44
- Whittaker, A. G. (1978). "Carbon: A New View of Its High-Temperature Behavior". Science. 200 (4343): 763–4. Bibcode:1978Sci...200..763G. doi:10.1126/science.200.4343.763. PMID 17743239. S2CID 45075306. As cited by Kroto(2010).
- Smith, P. P. K.; Buseck, P. R. (1982). "Carbyne Forms of Carbon: Do They Exist?". Science. 216 (4549): 984–6. Bibcode:1982Sci...216..984S. doi:10.1126/science.216.4549.984. PMID 17809068. S2CID 13290442. As cited by Kroto(2010).
- Chuan, Xu-yun; Want, Tong-kuan; Donnet, Jean-Baptiste (March 2005). "Stability and Existence of Carbyne with Carbon Chains" (PDF). New Carbon Materials. 20 (1): 83–92. Archived from the original (PDF) on 26 January 2016. Retrieved 22 January 2016.
- Kudryavtsev, Yu.P; Heimann R.B, P.B; Evsyukov, S.E (1996). "Carbynes:advances in the field of linear carbon-chain compounds". Journal of Materials Science. 31 (21): 5557–5571. Bibcode:1996JMatS..31.5557K. doi:10.1007/BF01160799. S2CID 95313003.
- Kudryavtsev, Yu.P; Evsyukov, S.E; Babaev, V.G (1992). "Oriented carbyne layers". Carbon. 30 (2): 213–221. doi:10.1016/0008-6223(92)90082-8.
- Goresy, A.E.; Donnat, G (1968). "Graphitic gneisses". Science. 161: 363.
- Kudryavtsev, Yu.P; Evsyukov, S.E; Guseva, M.B (1997). "Carbyne - a linear chainlike carbone allotrope". Chemistry and Physics of Carbon. 1: 2–70. doi:10.1201/9781482273199-8. ISBN 9780429182686.
- Whittaker, A.G (1979). "Carbon:occurrence of carbyne forms of carbon in natural graphite". Carbon. 17: 21–24. doi:10.1016/0008-6223(79)90066-6.
- E. A. Rohlfing; D. M. Cox; A. J. Kaldor (1984). "Production and characterization of supersonic carbon cluster beams". Journal of Chemical Physics. 81 (7): 3332. Bibcode:1984JChPh..81.3322R. doi:10.1063/1.447994. As cited by Kroto(2010).
- Yamada, K.; Kunishige, H.; Sawaoka, A. B. (1991). "Formation process of carbyne produced by shock compression". Naturwissenschaften. 78 (10): 450. Bibcode:1991NW.....78..450Y. doi:10.1007/BF01134379. S2CID 2504527.
- Lagow, R. J.; Kampa, J. J.; Wei, H. -C.; Battle, S. L.; Genge, J. W.; Laude, D. A.; Harper, C. J.; Bau, R.; Stevens, R. C.; Haw, J. F.; Munson, E. (1995). "Synthesis of Linear Acetylenic Carbon: The "sp" Carbon Allotrope". Science. 267 (5196): 362–367. Bibcode:1995Sci...267..362L. doi:10.1126/science.267.5196.362. PMID 17837484. S2CID 12939062.
- Kastner, J.; Kuzmany, H.; Kavan, L.; Dousek, F. P.; Kuerti, J. (1995). "Reductive Preparation of Carbyne with High Yield. An in Situ Raman Scattering Study". Macromolecules. 28 (1): 344–353. Bibcode:1995MaMol..28..344K. doi:10.1021/ma00105a048.
- Cataldo, Franco (1999). "From dicopper acetylide to carbyne". Polymer International. 48: 15–22. doi:10.1002/(SICI)1097-0126(199901)48:1<15::AID-PI85>3.0.CO;2-#.
- Cataldo, Franco (1997). "A study on the structure and electrical properties of the fourth carbon allotrope: Carbyne". Polymer International. 44 (2): 191–200. doi:10.1002/(SICI)1097-0126(199710)44:2<191::AID-PI842>3.0.CO;2-Y.
- Xue, K. H.; Tao, F. F.; Shen, W.; He, C. J.; Chen, Q. L.; Wu, L. J.; Zhu, Y. M. (2004). "Linear carbon allotrope – carbon atom wires prepared by pyrolysis of starch". Chemical Physics Letters. 385 (5–6): 477. Bibcode:2004CPL...385..477X. doi:10.1016/j.cplett.2004.01.007.
- "Route to Carbyne: Scientists Create Ultra-Long 1D Carbon Chains". Sci-news.com. 2016-04-09. Retrieved 2016-04-10.
- Shi, Lei; Rohringer, Philip; Suenaga, Kazu; Niimi, Yoshiko; Kotakoski, Jani; Meyer, Jannik C.; Peterlik, Herwig; Wanko, Marius; Cahangirov, Seymur; Rubio, Angel; Lapin, Zachary J.; Novotny, Lukas; Ayala, Paola; Pichler, Thomas (2016). "Confined linear carbon chains as a route to bulk carbyne". Nature Materials. 15 (6): 634–639. arXiv:1507.04896. Bibcode:2016NatMa..15..634S. doi:10.1038/nmat4617. PMID 27043782. S2CID 205413206.
- Chalifoux, W. A.; Tykwinski, R. R. (2009). "Synthesis of extended polyynes: Toward carbyne". Comptes Rendus Chimie. 12 (3–4): 341. doi:10.1016/j.crci.2008.10.004.
- Hadlington, Simon (19 September 2010). "One dimensional carbon chains get longer". Chemistry World. Royal Society of Chemistry.
- Eisler, S.; Slepkov, A. D.; Elliott, E.; Luu, T.; McDonald, R.; Hegmann, F. A.; Tykwinski, R. R. (2005). "Polyynes as a Model for Carbyne: Synthesis, Physical Properties, and Nonlinear Optical Response". Journal of the American Chemical Society. 127 (8): 2666–2676. doi:10.1021/ja044526l. PMID 15725024.
- Wanko, M; Cahangirov, Seymur; Shi, Lei; Rohringer, Philip; Lapin, Zachary J; Novotny, Lukas; Ayala, Paola; Pichler, Thomas; Rubio, Angel (2016). "Polyyne Electronic and Vibrational Properties under Environmental Interactions". Phys. Rev. B. 94 (19): 195422. arXiv:1604.00483. Bibcode:2016PhRvB..94s5422W. doi:10.1103/PhysRevB.94.195422.
- Emerging Technology From the arXiv August 15, 2013 (2013-08-15). "New Form of Carbon is Stronger Than Graphene and Diamond". MIT Technology Review. Retrieved 2013-12-24.
- "New one-dimensional form of carbon may be the strongest material ever". KurzweilAI. 11 October 2013.
- Liu, Mingjie; Artyukhov, Vasilii I.; Lee, Hoonkyung; Xu, Fangbo; Yakobson, Boris I. (2013). "Carbyne from first principles: Chain of C atoms, a nanorod or a nanorope". ACS Nano. 7 (11): 10075–82. arXiv:1308.2258. doi:10.1021/nn404177r. PMID 24093753. S2CID 23650957.
- Borghino, Dario (15 October 2013). "Carbyne: The new world's strongest material?". New Atlas.
- Sharma, Keshav; Costa, Nathalia (2020-08-31). "Anharmonicity and Universal Response of Linear Carbon Chain Mechanical Properties under Hydrostatic Pressure". Phys. Rev. Lett. 125 (10): 105051. Bibcode:2020PhRvL.125j5501S. doi:10.1103/PhysRevLett.125.105501. hdl:1721.1/132299. PMID 32955330. S2CID 221828448.
- Shi, Lei; Rohringer, Philip; Wanko, Marius; Rubio, Angel; Waßerroth, Sören; Reich, Stephanie; Cambré, Sofie; Wenseleers, Wim; Ayala, Paola; Pichler, Thomas (2016). "Electronic band gaps of confined linear carbon chains ranging from polyyne to carbyne". Physical Review Materials. 1 (7): 075601. doi:10.1103/PhysRevMaterials.1.075601. hdl:21.11116/0000-0001-6B23-0. S2CID 119087831.
- Rohringer, Philip; Shi, Lei; Ayala, Paola; Pichler, Thomas (2016). "Selective Enhancement of Inner Tube Photoluminescence in Filled Double-Walled Carbon Nanotubes". Advanced Functional Materials. 26 (27): 4874–4881. doi:10.1002/adfm.201505502.
- Sorokin, Pavel B.; Lee, Hoonkyung; Antipina, Lyubov Yu.; Singh, Abhishek K.; Yakobson, Boris I. (2011). "Calcium-decorated carbyne networks as hydrogen storage media". Nano Letters. 11 (7): 2660–2665. Bibcode:2011NanoL..11.2660S. doi:10.1021/nl200721v. PMID 21648444.
- Tschannen, Cla Duri; Gordeev, Georgy; Reich, Stephanie; Shi, Lei; Pichler, Thomas; Frimmer, Martin; Novotny, Lukas; Heeg, Sebastian (2020). "Raman Scattering Cross Section of Confined Carbyne". Nano Letters. 20 (9): 6750–6755. Bibcode:2020NanoL..20.6750T. doi:10.1021/acs.nanolett.0c02632. PMID 32786933. S2CID 220055830.
Further reading
- Diederich, François; Stang, Peter J.; Tykwinski, R. R. (Rik R.), eds. (2005). "Chapter 9. Carbon-Rich Compounds: Acetylene-Based Carbon Allotropes". Acetylene chemistry : chemistry, biology, and material science. Weinheim: Wiley-VCH. pp. 387–426. ISBN 978-3527307814. OCLC 57483840.