Sponge spicule
Spicules are structural elements found in most sponges. The meshing of many spicules serves as the sponge's skeleton and thus it provides structural support and potentially defense against predators.[1]
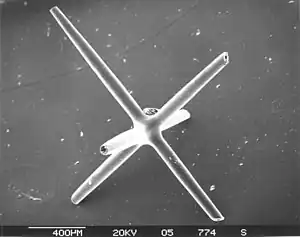
.jpg.webp)
similar spicules
Sponge spicules are made of calcium carbonate or silica. Large spicules visible to the naked eye are referred to as megascleres or macroscleres, while smaller, microscopic ones are termed microscleres. The composition, size, and shape of spicules are major characters in sponge systematics and taxonomy.
Overview
Part of a series related to |
Biomineralization |
---|
![]() |
|

Sponges are a species-rich clade of the earliest-diverging (most basal) animals.[3] They are distributed globally,[4] with diverse ecologies and functions,[5][6][7][8][9][10] and a record spanning at least the entire Phanerozoic.[11]
Most sponges produce skeletons formed by spicules, structural elements that develop in a wide variety of sizes and three dimensional shapes. Among the four sub-clades of Porifera, three (Demospongiae, Hexactinellida, and Homoscleromorpha) produce skeletons of amorphous silica [12] and one (Calcarea) of magnesium-calcite.[13] It is these skeletons that are composed of the elements called spicules.[14][15] The morphologies of spicules are often unique to clade- or even species-level taxa, and this makes them useful in taxonomic assignments.[16]
Research history
In 1833, Robert Edmond Grant grouped sponges into a phylum he called Porifera (from the Latin porus meaning "pore" and -fer meaning "bearing").[17] He described sponges as the simplest of multicellular animals, sessile, marine invertebrates built from soft, spongy (amorphously shaped) material.[17]
Later, the Challenger expedition (1873–1876) discovered deep in the ocean a rich collection of glass sponges (class Hexactinellida), which radically changed this view. These glass sponges were described by Franz Schulze (1840–1921), and came to be regarded as strongly individualised radially symmetric entities representing the phylogenetically oldest class of siliceous sponges.[18] They are eye-catching because of their distinct body plan (see lead image above) which relies on a filigree skeleton constructed using an array of morphologically determined spicules.[19]
Then, during the German Deep Sea Expedition "Valdivia" (1898-1899), Schulze described the largest known siliceous hexactinellid sponge, the up to three metres high Monorhaphis chuni. This sponge develops the also largest known bio-silicate structures, giant basal spicules, three metres high and one centimetre thick. With such spicules as a model, basic knowledge on the morphology, formation, and development of the skeletal elements could be elaborated. Spicules are formed by a proteinaceous scaffold which mediates the formation of siliceous lamellae in which the proteins are encased. Up to eight hundred 5 to 10 μm thick lamellae can be concentrically arranged around an axial canal. The silica matrix is composed of almost pure silicon and oxygen, providing it with unusual optophysical properties superior to man-made waveguides.[19]
Since their discovery, hexactinellids were appraised as "the most characteristic inhabitants of the great depths", rivalling in beauty the other class of siliceous Porifera, the demosponges.[20] Their thin network of living tissues is supported by a characteristic skeleton, a delicate scaffold of siliceous spicules, some of which may be fused together by secondary silica deposition to form a rigid framework.[21] The Hexactinellida together with the Demospongiae forms a common taxonomic unit comprising the siliceous sponges. The spicules, the elements from which their skeletons are constructed, are built in a variety of distinct shapes, and are made from silica that is deposited in the form of amorphous opal (SiO2·nH2O).[19]
In evolution, after the Ediacaran period, a third class of Porifera appeared, the Calcarea, which has a calcium-carbonate skeleton.[22][19]
Sponges have been receiving special attention from researchers since the introduction of molecular biological techniques at the turn of the century, since findings point to sponges as the phylogenetically oldest animal phylum.[19] New information has accumulated concerning the relevance of this phylum for understanding of the dynamics of evolutionary processes that occurred during the Ediacaran, the time prior to the Cambrian Explosion which can be dated back to approximately 540 million years ago.[19] According to molecular data from sponge genes that encode receptors and signal transduction molecules,[23][24] the Hexactinellida were established to be the phylogenetically oldest class of the Porifera. Based on the discovery that the Porifera share one common ancestor, the Urmetazoa, with the other animals,[25][26] it was deduced that these animals represent the oldest, still extant animal taxon. Even more, the emergence of these animals could be calculated back to 650–665 million years ago [Ma], a date that was confirmed by fossils records.[11] Hence the Porifera must have lived already prior to the Ediacaran-Cambrian boundary, 542 Ma, and thus their elucidated genetic toolkit may contribute to the understanding of the Ediacaran soft-bodied biota as well, as sketched by Pilcher.[27] It was the evolutionary novelty, the formation of a hard skeleton, that contributed significantly to the radiation of the animals in the late Proterozoic [28] and the construction of the metazoan body plan.[29]
Spicule types
Sponge spicules can be calcareous or siliceous. Siliceous spicules are sometimes embedded in spongin. Spicules are found in a range of symmetry types.
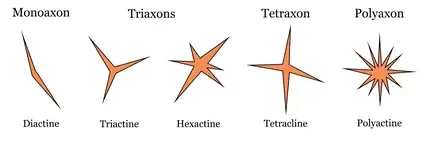
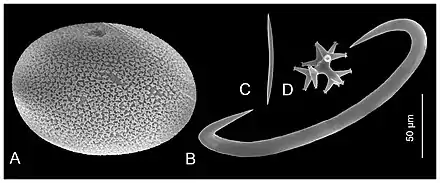
Monaxons form simple cylinders with pointed ends. The ends of diactinal monaxons are similar, whereas monactinal monaxons have different ends: one pointed, one rounded. Diactinal monaxons are classified by the nature of their ends: oxea have pointed ends, and strongyles are rounded. Spine-covered oxea and strongyles are termed acanthoxea and acanthostrongyles, respectively.[31]: 2 Monactical monaxons always have one pointed end; they are termed styles if the other end is blunt, tylostyles if their blunt end forms a knob; and acanthostyles if they are covered in spines.
Triaxons have three axes; in triods, each axis bears a similar ray; in pentacts, the triaxon has five rays, four of which lie in a single plane; and pinnules are pentacts with large spines on the non-planar ray.[31]
Tetraxons have four axes, and polyaxons more (description of types to be incorporated from [31]). Sigma-C spicules have the shape of a C.[31]
Dendroclones might be unique to extinct sponges[32] and are branching spicules that may take irregular forms, or may form structures with an I, Y or X shape.[33][34]
- Megascleres are large spicules measuring from 60-2000 μm and often function as the main support elements in the skeleton.[35]
- Acanthostyles are spiny styles.
- Anatriaenes, orthotriaenes and protriaenes are triaenes[36] - megascleres with one long and three short rays.
- Strongyles are megascleres with both ends blunt or rounded.
- Styles are megascleres with one end pointed and the other end rounded.
- Tornotes are megascleres with spear shaped ends.
- Tylotes are megascleres with knobs on both ends.
- Microscleres are small spicules measuring from 10-60 μm and are scattered throughout the tissue and are not part of the main support element.[35]
- Chelae are microscleres with shovel-like structures on the ends. Anisochelas are microscleres with dissimilar ends. Isochelas are microscleres with two similar ends.
- Euasters are star-shaped microscleres with multiple rays radiating from a common centre. Examples are oxyasters (euasters with pointed rays) or sterrasters (ball-shaped euasters).
- Forceps are microscleres bent back on themselves.
- Microstrongyles are small rods with both ends blunt or rounded.
- Microxeas are small rods with both ends pointed.
- Sigmas are "C" or "S" shaped microscleres.
Calcareous spicules
Animal biomineralization is a controlled process and leads to the production of mineral–organic composite materials that considerably differ in shape and material properties from their purely inorganic counterparts. The ability to form functional biominerals, such as endoskeletons and exoskeletons, protective shells, or teeth, had been a significant step in animal evolution. Calcium carbonate biomineralization, the most widespread type among animal phyla,[37] evolved several times independently, resulting in multiple recruitments of the same genes for biomineralization in different lineages.[38]
Among these genes, members of the alpha carbonic anhydrase gene family (CAs) are essential for biomineralization.[39] CAs are zinc-binding enzymes that catalyze the reversible conversion of carbon dioxide and water to bicarbonate and one proton.[40] The zinc-binding is mediated by three histidine residues essential for the protein's catalytic function.[41][42] CAs are involved in many physiological processes requiring ion regulation or carbon transport,[43] both of which are crucial for the controlled precipitation of carbonate biominerals. In mammals, where they are best studied, 16 different CAs are expressed in specific tissues and active in defined subcellular compartments.[44] Cytosolic, mitochondrial, membrane-bound, and secreted CA forms can be distinguished, and these groups got expanded and reduced in different animal groups.[39][45] Specific CAs are involved in the carbonate biomineralization in distinct animal lineages,[39] including sponges.[46][45][47][48]
Among extant sponges, only the calcareous sponges can produce calcite spicules, whereas other classes' spicules are siliceous. Some lineages among demosponges and a few calcareans have massive calcium carbonate basal skeletons, the so-called coralline sponges or sclerosponges. The biomineralizing CAs used by carbonate-producing demosponges are not orthologous to the CAs involved in the spicule formation of calcareous sponges,[45] suggesting that the two biomineralization types evolved independently. This observation agrees with the idea that the formation of calcitic spicules is an evolutionary innovation of calcareous sponges.[49][48]
Spicules are formed by sclerocytes, which are derived from archaeocytes. The sclerocyte begins with an organic filament, and adds silica to it. Spicules are generally elongated at a rate of 1-10 μm per hour. Once the spicule reaches a certain length it protrudes from the sclerocyte cell body, but remains within the cell's membrane. On occasion, sclerocytes may begin a second spicule while the first is still in progress.[51]
The shapes of calcareous sponge spicules are simple compared with the sometimes very elaborate siliceous spicules found in the other sponge classes. With only a few exceptions, calcareous sponge spicules can be of three basic types: monaxonic, two-tipped diactines, triactines with three spicules rays, and four-rayed tetractines. Specialized cells, the sclerocytes, produce these spicules, and only a few sclerocytes interact in the formation of one specific spicule: Two sclerocytes produce a diactine, six sclerocytes form a triactine, and seven a tetractines.[52][53][54] A pair of sclerocytes is involved in the growth of each actine of these spicules. After an initial phase, the so-called founder cell promotes actine elongation, the second, so-called thickener cell in some, but not all species deposit additional calcium carbonate on the actine, as it migrates back toward the founder cell.[54][55] Calcareous sponges can possess only one or any combination of the three spicule types in their body, and in many cases, certain spicule types are restricted to specific body parts. This indicates that spicule formation is under strict genetic control in calcareous sponges, and specific CAs play an essential role in this genetic control[50][48]
Siliceous spicules
.jpg.webp)
Shown left: The largest biosilica structure on Earth is the giant basal spicule from the deep-sea glass sponge Monorhaphis chuni.[19]
The largest biosilica structure on Earth is the giant basal spicule from the deep-sea glass sponge Monorhaphis chuni.[19] The diagram on the right shows:
- (a) Young specimens of M. chuni anchored to the muddy substratum by one single giant basal spicule (gbs). The body (bo) surrounds the spicule as a continuous, round cylinder.
- (b) The growth phases of the sessile animal with its GBS (gbs) which anchors it to the substratum and holds the surrounding soft body (bo). The characteristic habitus displays linearly arranged large atrial openings (at) of approximately 2 cm in diameter. With growth, the soft body dies off in the basal region and exposes the bare GBS (a to c).
- (c) Part of the body (bo) with its atrial openings (at). The body surface is interspersed with ingestion openings allowing a continuous water flow though canals in the interior which open into oscules that are centralized in atrial openings, the sieve-plates.
- (d) M. chuni in its natural soft bottom habitat of bathyal slopes off New Caledonia. The specimens live at a depth of 800–1,000 m [23]. In this region, the sponge occurs at a population density of 1-2 individuals per m2. The animals reach sizes of around 1 m in length.
- (e) Drawings of different glass sponges (hexactinellids).[19]
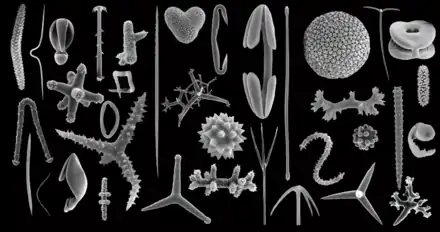
Not to scale — sizes vary between 0.01 and 1 mm
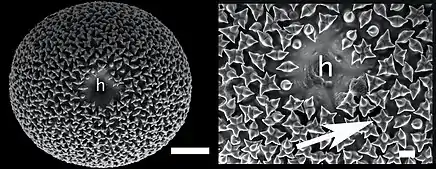
Geodia atlantica; (h) is the hilum; the arrow points to a smooth rosette made up of rays. Left scale 20 μm, right scale 2 μm.

Geodia macandrewii, left scale 50 μm, right scale 2 μm
Siliceous spicules in demosponges exist in a variety of shapes, some of which look like minute spheres of glass. They are called sterrasters when they belong to the Geodiidae family and selenasters when they belong to the Placospongiidae family.[58]
Siliceous spicules were first described and illustrated in 1753 by Vitaliano Donati,[59] who found them in the species Geodia cydonium from the Adriatic Sea: he called these spicules "little balls". They are later called globular crystalloids, globate spicules, or globostellates by sponge taxonomists, until 1888 when William Sollas [60] finally coins the term "sterraster" from the Greek sterros meaning "solid" or "firm" – see diagram on the right. Meanwhile, similar ball-shaped spicules are observed in another genus, Placospongia, and these are at first considered as "sterrasters" [60] before Richard Hanitsch coins the term "selenaster" in 1895 [61] for these different spicules (coming from the Greek selene for "moon", referring to the "half-moon" shape). Finally, an additional term "aspidaster" is created by von Lendenfeld in 1910,[62] convinced that the flattened sterrasters in the genus Erylus are significantly different from those in Geodia.[58]
Today, the Geodiidae represent a highly diverse sponge family with more than 340 species, occurring in shallow to deep waters worldwide apart from the Antarctic. Sterrasters/aspidaster spicules are currently the main synapomorphy of the Geodiidae. The family currently includes five genera with sterrasters and several others that have secondarily lost their sterrasters.[63][64] The Geodia can be massive animals more than a meter across.[65][66][67][58]
Selenasters are the main synapomorphy of Placospongia (family Placospongiidae, order Clionaida), a well-supported monophyletic genus [68] from shallow temperate/tropical waters worldwide. It is not a very diverse genus with only 10 species currently described (WPD) and a handful of undescribed species.[69][68] Placospongia species are usually small, encrusting, and never occur in high densities.[58]
Sterrasters/selenasters are big enough to examine in some detail their surfaces with an optical microscope. However, the use of the scanning electron microscope (SEM) enabled a significantly better understanding of the surface microornamentations. A few descriptive terms have also appeared to describe and compare in greater detail the microornamentations of these ball-shaped spicules. polyaxial spicules such as the sterrasters and aspidasters, are the result of fused "actines" (= branches of asters, from the Greek for "star"), later covered with "rosettes" made of different "rays". The "hilum" (Latin for a "little thing" or "trifle" or the "eye of a bean") is a small area without rosettes or any kind of surface pattern. There are no particular terms to describe the surface of selenasters, except for the "hilum", also present. Although there appears to be no significant variation in the size of the rosettes and hilum between species,[70][67] noticed that rosettes could be smooth or warty and hypothesized that this character could be of phylogenetic value if studied more broadly. Furthermore, the rosette morphology also seemed to be variable between Geodia, Pachymatisma, and Caminella [71][72] which suggests that a more detailed study of the sterraster/aspidaster surface would potentially bring new characters for Geodiidae genera identification.[58]
Spicule "life cycle"
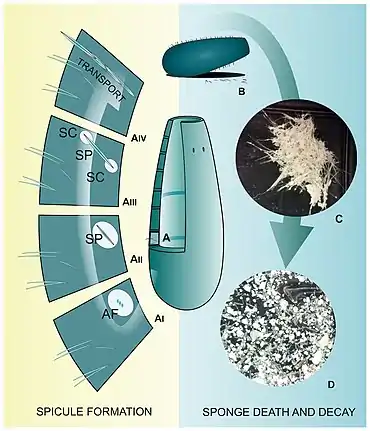
From formation to deposition
The formation of spicules is controlled genetically.[73] In most cases, the first growth phase is intracellular; it starts in sclerocytes (amoeboid cells responsible for spicule formation) in mesohyl [74][75] and is mediated by silicatein, a special enzyme that initiates formation of the axial filament (harboured by the axial canal) which provides the vertical axis of the spicule.[76] The axial canal is filled with organic proteinaceous material which usually extends to the tip of the newly-formed spicule.[77] The cross-section of the axial canal differs across major sponge clades that produce siliceous spicules (it is triangular in demosponges,[78] irregular in homoscleromorphs [14] and quadrangular in hexactinellids.[79] In calcareans (producing calcareous spicules) the axial canal is not developed.[79] The geometry and the length of the axial filament determines the shape of the spicule.[80] In desmoid spicules of 'lithistids' (an informal group of demosponges with articulated skeletons), however, the axial filament is shorter than the spicule arms and it is possible that only organic molecules are involved in the spicule-forming process.[80][16]
During formation of the siliceous spicules (Calcarea displays different mechanisms of spicule biomineralization), sponges obtain silicon in the form of soluble silicic acid and deposit it around the axial filament, [78][14] within a special membrane called silicalemma.[81][82] Silica is first laid out as small 2 μm granules [78][80] that are fused to bigger spheres (or fused together within process of biosintering in Hexactinellida.[83] After some time, amorphous silica is added, forming evenly-deposited concentric layers,[14] separated from each other by ultrathin organic interlayers.[84] At this stage, immature spicules are secreted from the sclerocyte and covered by pseudopodia of one to several cells, and the process of silica deposition and spicule growth continues.[78][16]
After completing the deposition of silica (or during this phase), the spicule is transported to the right place in the sponge body by crawling mesohyl cells, where spongocytes secrete spongin fibrils around them and connect them with adjacent spicules.[14] In some hexactinellids, that are characterized by rigid skeleton, the fusion of spicules appears to occur parallel to spicule secretion.[85][16]
When sponges are alive, their spicules provide a structural "framework". Following their death, the body and the skeleton structure, especially that of demosponges in which the spicules are connected to each other only by perishable collagen fibres, rapidly disintegrate leaving the spicules "free". Because of this, sponges are rarely wholly preserved in the fossil record. Their spicules, however, are incorporated into sediments, often becoming one of the main components of sedimentary rocks.[86][87] Sometimes spicules accumulate into enormous agglomerations called spicule mats or beds.[88] These accumulations are characteristic for polar waters.[89][90] Spicules can fossilize to form special type of rocks called the spiculites ("spongillites" for freshwater sponge spicules); these types of rocks are known globally,[91][92][93][94] and have been formed through the whole Phanerozoic.[92] Biosiliceous sedimentation occasionally results in the formation of spiculitic cherts (in so called glass ramps) which are recorded from the Permian to Eocene of many parts of the world.[95][96][16]
Locomotion
In 2016 a newly discovered demosponge community living under arctic ice were found to have moved across the sea floor by extending their spicules and then retracting their body in the direction of motion.[97]
Spiculites
When dead sponge bodies disintegrate, spicules become incorporated into marine sediments and sometimes accumulate into enormous agglomerations called spicule mats or beds, or fossilize to form special type of rocks called the spiculites.[16]
The record of fossil and subfossil sponge spicules is extraordinarily rich and often serves as a basis for far-reaching reconstructions of sponge communities, though spicules are also bearers of significant ecological and environmental information. Specific requirements and preferences of sponges can be used to interpret the environment in which they lived, and reconstruct oscillations in water depths, pH, temperatures, and other parameters, providing snapshots of past climate conditions. In turn, the silicon isotope compositions in spicules (δ30Si) are being increasingly often used to estimate the level of silicic acid in the marine settings throughout the geological history, which enables the reconstruction of past silica cycle and ocean circulation.[16]
Spicules provide structural support for maintaining the vertical body position, minimize the metabolic cost of water exchange, [98][14] and may even deter predators.[14] They often develop in different sizes [12] and a wide variety of three dimensional shapes, with many being unique to clade- or even species-level taxa. Demosponges are characterized by spicules of monaxonic or tetraxonic symmetry.[12] Hexactinellids produce spicules of hexactinic or triaxonic (cubic) symmetry or shapes that are clearly derived from such morpohologies.[21] The spicules of homoscleromorphs represent peculiar tetractines (calthrops) and their derivatives that originate through reduction or ramification of the clads.[99] Spicules of Calcarea are produced in three basic forms: diactines, triactines and tetractines.[100][16]
The mineral composition of sponge spicules makes these structures the most resistant parts of the sponge bodies [79] and ensures the ability of spicules to withstand various taphonomic processes,[86][101] resulting in that they often constitute the only evidence of the presence of some sponges in an ecosystem.[102] Even though sponges are often known from rich assemblages of bodily-preserved specimens,[103][104][105] a significant part of their fossil and subfossil record is also represented by their spicules. Having that in mind, spicules can be of crucial importance for reconstructions of extinct or cryptic (hiding in cervices and caves) sponge communities; and, indeed, they have been investigated especially with respect to their taxonomic significance.[106][12] The morphologies of spicules and their arrangement, together with other important sponge features, such as the shape, consistency, and color, are essential when identifying sponges.[107][16]
In contrast to whole-bodied sponge fossils, spicules are common in many depositional environments.[108] Their significance, however, is often underestimated, which is mostly due to the difficulties in assigning disassociated spicules to sponge taxa or due to the scarcity of the material.[16]
Interaction with light
Research on the Euplectella aspergillum (Venus' Flower Basket) demonstrated that the spicules of certain deep-sea sponges have similar traits to Optical fibre. In addition to being able to trap and transport light, these spicules have a number of advantages over commercial fibre optic wire. They are stronger, resist stress easier, and form their own support elements. Also, the low-temperature formation of the spicules, as compared to the high temperature stretching process of commercial fibre optics, allows for the addition of impurities which improve the refractive index. In addition, these spicules have built-in lenses in the ends which gather and focus light in dark conditions. It has been theorized that this ability may function as a light source for symbiotic algae (as with Rosella racovitzae) or as an attractor for shrimp which live inside the Venus' Flower Basket. However, a conclusive decision has not been reached; it may be that the light capabilities are simply a coincidental trait from a purely structural element. [51][109][110] Spicules funnel light deep inside sea sponges.[111][112]
- Sponge spicules
- Spicules of sponge (SEM)
- Network of sponge spicules
See also
References
- Jones, Adam C.; Blum, James E.; Pawlik, Joseph R. (2005-08-08). "Testing for defensive synergy in Caribbean sponges: Bad taste or glass spicules?". Journal of Experimental Marine Biology and Ecology. 322 (1): 67–81. doi:10.1016/j.jembe.2005.02.009. ISSN 0022-0981. S2CID 85614908.
- Riesgo, Ana; Taboada, Sergi; Kenny, Nathan J.; Santodomingo, Nadia; Moles, Juan; Leiva, Carlos; Cox, Eileen; Avila, Conxita; Cardona, Luis; Maldonado, Manuel (2021). "Recycling resources: silica of diatom frustules as a source for spicule building in Antarctic siliceous demosponges". Zoological Journal of the Linnean Society. 192 (2): 259–276. doi:10.1093/zoolinnean/zlaa058.
- Wörheide, G.; Dohrmann, M.; Erpenbeck, D.; Larroux, C.; Maldonado, M.; Voigt, O.; Borchiellini, C.; Lavrov, D.V. (2012). "Deep Phylogeny and Evolution of Sponges (Phylum Porifera)". Advances in Sponge Science: Phylogeny, Systematics, Ecology. Advances in Marine Biology. Vol. 61. pp. 1–78. doi:10.1016/B978-0-12-387787-1.00007-6. ISBN 9780123877871. PMID 22560777.
- Van Soest, Rob W. M.; Boury-Esnault, Nicole; Vacelet, Jean; Dohrmann, Martin; Erpenbeck, Dirk; De Voogd, Nicole J.; Santodomingo, Nadiezhda; Vanhoorne, Bart; Kelly, Michelle; Hooper, John N. A. (2012). "Global Diversity of Sponges (Porifera)". PLOS ONE. 7 (4): e35105. Bibcode:2012PLoSO...735105V. doi:10.1371/journal.pone.0035105. PMC 3338747. PMID 22558119.
- Wulff, Janie L. (1984). "Sponge-mediated coral reef growth and rejuvenation". Coral Reefs. 3 (3): 157–163. Bibcode:1984CorRe...3..157W. doi:10.1007/BF00301960. S2CID 41590456.
- Wulff, Janie L. (2006). "Rapid diversity and abundance decline in a Caribbean coral reef sponge community". Biological Conservation. 127 (2): 167–176. doi:10.1016/j.biocon.2005.08.007.
- Bell, James J. (2008). "The functional roles of marine sponges". Estuarine, Coastal and Shelf Science. 79 (3): 341–353. Bibcode:2008ECSS...79..341B. doi:10.1016/j.ecss.2008.05.002.
- Maldonado, Manuel; Ribes, Marta; Van Duyl, Fleur C. (2012). "Nutrient Fluxes Through Sponges". Advances in Sponge Science: Physiology, Chemical and Microbial Diversity, Biotechnology. Advances in Marine Biology. Vol. 62. pp. 113–182. doi:10.1016/B978-0-12-394283-8.00003-5. ISBN 9780123942838. PMID 22664122.
- De Goeij, Jasper M.; Van Oevelen, Dick; Vermeij, Mark J. A.; Osinga, Ronald; Middelburg, Jack J.; De Goeij, Anton F. P. M.; Admiraal, Wim (2013). "Surviving in a Marine Desert: The Sponge Loop Retains Resources within Coral Reefs". Science. 342 (6154): 108–110. Bibcode:2013Sci...342..108D. doi:10.1126/science.1241981. PMID 24092742. S2CID 6720678.
- De Goeij, Jasper M.; Lesser, Michael P.; Pawlik, Joseph R. (2017). "Nutrient Fluxes and Ecological Functions of Coral Reef Sponges in a Changing Ocean". Climate Change, Ocean Acidification and Sponges (PDF). pp. 373–410. doi:10.1007/978-3-319-59008-0_8. hdl:11245.1/97acf693-165b-485e-aa13-624af511bccd. ISBN 978-3-319-59007-3.
- Reitner, Joachim; Wörheide, Gert (2002). "Non-Lithistid Fossil Demospongiae — Origins of their Palaeobiodiversity and Highlights in History of Preservation". Systema Porifera. pp. 52–68. doi:10.1007/978-1-4615-0747-5_4. ISBN 978-0-306-47260-2.
- Hooper, John N. A.; Van Soest, Rob W. M. (2002). "Systema Porifera. A Guide to the Classification of Sponges". Systema Porifera. pp. 1–7. doi:10.1007/978-1-4615-0747-5_1. ISBN 978-0-306-47260-2.
- Rossi, Andre L.; Campos, Andrea P.C.; Barroso, Madalena M.S.; Klautau, Michelle; Archanjo, Bráulio S.; Borojevic, Radovan; Farina, Marcos; Werckmann, Jacques (2014). "Long-range crystalline order in spicules from the calcareous sponge Paraleucilla magna (Porifera, Calcarea)". Acta Biomaterialia. 10 (9): 3875–3884. doi:10.1016/j.actbio.2014.01.023. PMID 24487057.
- Uriz, María-J.; Turon, Xavier; Becerro, Mikel A.; Agell, Gemma (2003). "Siliceous spicules and skeleton frameworks in sponges: Origin, diversity, ultrastructural patterns, and biological functions". Microscopy Research and Technique. 62 (4): 279–299. doi:10.1002/jemt.10395. PMID 14534903. S2CID 535640.
- Sethmann, Ingo; Wörheide, Gert (2008). "Structure and composition of calcareous sponge spicules: A review and comparison to structurally related biominerals". Micron. 39 (3): 209–228. doi:10.1016/j.micron.2007.01.006. PMID 17360189.
- Łukowiak, Magdalena (2020). "Utilizing sponge spicules in taxonomic, ecological and environmental reconstructions: A review". PeerJ. 8: e10601. doi:10.7717/peerj.10601. PMC 7751429. PMID 33384908.
Material was copied from this source, which is available under a Creative Commons Attribution 4.0 International License.
- Grant, R. E. (1833). "Lectures on comparative anatomy and animal physiology; on the classification of the animals". The Lancet. 1: 153–159.
- Hyman, L. H. (1992). The Invertebrates: Protozoa Through Ctenophora. International Books & Periodicals Supply Service. ISBN 9780785503712.
- Wang, Xiaohong; Gan, Lu; Jochum, Klaus P.; Schröder, Heinz C.; Müller, Werner E. G. (2011). "The Largest Bio-Silica Structure on Earth: The Giant Basal Spicule from the Deep-Sea Glass Sponge Monorhaphis chuni". Evidence-Based Complementary and Alternative Medicine. 2011: 1–14. doi:10.1155/2011/540987. PMC 3166767. PMID 21941585.
Material was copied from this source, which is available under a Creative Commons Attribution 4.0 International License.
- Campbell, Lord George Granville (21 February 2018). Log-Letters from the Challenger: By Lord George Campbell. Creative Media Partners, LLC. ISBN 9781378320709.
- Leys, S.P.; MacKie, G.O.; Reiswig, H.M. (2007). The Biology of Glass Sponges. Advances in Marine Biology. Vol. 52. pp. 1–145. doi:10.1016/S0065-2881(06)52001-2. ISBN 9780123737182. PMID 17298890.
- Schütze, Joachim; Krasko, Anatoli; Custodio, Marcio Reis; Efremova, Sofla M.; Müller, Isabel M.; Müller, Werner E. G. (1999-01-07). "Evolutionary relationships of Metazoa within the eukaryotes based on molecular data from Porifera". Proceedings of the Royal Society of London. Series B: Biological Sciences. The Royal Society. 266 (1414): 63–73. doi:10.1098/rspb.1999.0605. ISSN 0962-8452. PMC 1689648. PMID 10081159.
- Kruse, M.; Muller, I. M.; Muller, W. E. (1997). "Early evolution of metazoan serine/Threonine and tyrosine kinases: Identification of selected kinases in marine sponges". Molecular Biology and Evolution. 14 (12): 1326–1334. doi:10.1093/oxfordjournals.molbev.a025742. PMID 9402742.
- Kruse, Michael; Leys, Sally P.; Müller, Isabel M.; Müller, Werner E.G. (1998). "Phylogenetic Position of the Hexactinellida within the Phylum Porifera Based on the Amino Acid Sequence of the Protein Kinase C from Rhabdocalyptus dawsoni". Journal of Molecular Evolution. 46 (6): 721–728. Bibcode:1998JMolE..46..721K. doi:10.1007/PL00006353. PMID 9608055. S2CID 10557258.
- Müller, Werner E. G. (1998). "Origin of Metazoa: Sponges as Living Fossils". Naturwissenschaften. 85 (1): 11–25. Bibcode:1998NW.....85...11M. doi:10.1007/s001140050444. PMID 9484707. S2CID 27535038.
- Müller, W. E. G.; Müller, I. M.; Rinkevich, B.; Gamulin, V. (1995). "Molecular evolution: Evidence for the monophyletic origin of multicellular animals". Naturwissenschaften. 82 (1): 36–38. Bibcode:1995NW.....82...36M. doi:10.1007/BF01167869. PMID 7898582. S2CID 29453726.
- Pilcher, Helen (2005). "Back to our roots". Nature. 435 (7045): 1022–1023. Bibcode:2005Natur.435.1022P. doi:10.1038/4351022a. PMID 15973376. S2CID 28844289.
- Knoll, A. H. (2003). "Biomineralization and Evolutionary History". Reviews in Mineralogy and Geochemistry. 54 (1): 329–356. Bibcode:2003RvMG...54..329K. doi:10.2113/0540329.
- "Molecular Morphogenesis: Gene Expression Patterns in Animals". Reviews in Cell Biology and Molecular Medicine. 2 (1): 1–41. 2016. doi:10.1002/3527600906.mcb.200500041.pub2 (inactive 1 August 2023). ISBN 9783527600908.
{{cite journal}}
: CS1 maint: DOI inactive as of August 2023 (link) - Pier, J. Q. (2019) "Porifera" Digital Atlas of Ancient Life, Paleontological Research Institution, New York. Updated: 16 Sept 2020.
- Eugenio Andri; Stefania Gerbaudo; Massimiliano Testa (2001). "13. Quaternary siliceous sponge spicules in the western Woodlark basin, southwest Pacific (ODP LEG 180)" (PDF). In Huchon, P.; Taylor, B.; Klaus, A (eds.). Proceedings of the Ocean Drilling Program, Scientific Results. Vol. 180. pp. 1–8.
- Komal Sutar and Bhavika Bhoir. "Study of the sponge spicules from the coastline of Raigad district, Maharashtra, India" (PDF). vpmthane.org/.
- Rigby, J. K.; Boyd, D. W. (2004). "Sponges from the Park City Formation (Permian) of Wyoming". Journal of Paleontology. 78: 71–76. doi:10.1666/0022-3360(2004)078<0071:SFTPCF>2.0.CO;2. ISSN 0022-3360. S2CID 130654425.
- Bingli, L.; Rigby, J.; Zhongde, Z. (2003). "Middle Ordovician Lithistid Sponges from the Bachu-Kalpin Area, Xinjiang, Northwestern China". Journal of Paleontology. Paleontological Society. 77 (3): 430–441. doi:10.1666/0022-3360(2003)077<0430:MOLSFT>2.0.CO;2. JSTOR 4094792. S2CID 130617208.
- Boury-Esnault, Nicole; Rützler, Klaus (1997). "Thesaurus of Sponge Morphology". Smithsonian Contributions to Zoology. 596 (596): 1–55. doi:10.5479/si.00810282.596.
- Boury-Esnault, Nicole, and Klaus Rutzler, editors. Thesaurus of Sponge Morphology. Smithsonian Contributions to Zoology, number 596, 55 pages, 305 figures, 1997. https://www.portol.org/thesaurus
- Murdock, Duncan J.E.; Donoghue, Philip C.J. (2011). "Evolutionary Origins of Animal Skeletal Biomineralization". Cells Tissues Organs. 194 (2–4): 98–102. doi:10.1159/000324245. PMID 21625061. S2CID 45466684.
- Murdock, Duncan J. E. (2020). "The 'biomineralization toolkit' and the origin of animal skeletons". Biological Reviews. 95 (5): 1372–1392. doi:10.1111/brv.12614. PMID 32447836. S2CID 218872873.
- Le Roy, Nathalie; Jackson, Daniel J.; Marie, Benjamin; Ramos-Silva, Paula; Marin, Frédéric (2014). "The evolution of metazoan α-carbonic anhydrases and their roles in calcium carbonate biomineralization". Frontiers in Zoology. 11. doi:10.1186/s12983-014-0075-8. S2CID 4846408.
- Tripp, Brian C.; Smith, Kerry; Ferry, James G. (2001). "Carbonic Anhydrase: New Insights for an Ancient Enzyme". Journal of Biological Chemistry. 276 (52): 48615–48618. doi:10.1074/jbc.r100045200. PMID 11696553.
- Aspatwar, Ashok; Tolvanen, Martti E. E.; Ortutay, Csaba; Parkkila, Seppo (2014). "Carbonic Anhydrase Related Proteins: Molecular Biology and Evolution". Carbonic Anhydrase: Mechanism, Regulation, Links to Disease, and Industrial Applications. Subcellular Biochemistry. Vol. 75. pp. 135–156. doi:10.1007/978-94-007-7359-2_8. ISBN 978-94-007-7358-5. PMID 24146378.
- Kim, Jin Kyun; Lee, Cheol; Lim, Seon Woo; Adhikari, Aniruddha; Andring, Jacob T.; McKenna, Robert; Ghim, Cheol-Min; Kim, Chae Un (2020). "Elucidating the role of metal ions in carbonic anhydrase catalysis". Nature Communications. 11 (1): 4557. Bibcode:2020NatCo..11.4557K. doi:10.1038/s41467-020-18425-5. PMC 7486293. PMID 32917908.
- Supuran, Claudiu T. (2016). "Structure and function of carbonic anhydrases". Biochemical Journal. 473 (14): 2023–2032. doi:10.1042/bcj20160115. PMID 27407171.
- Imtaiyaz Hassan, Md.; Shajee, Bushra; Waheed, Abdul; Ahmad, Faizan; Sly, William S. (2013). "Structure, function and applications of carbonic anhydrase isozymes". Bioorganic & Medicinal Chemistry. 21 (6): 1570–1582. doi:10.1016/j.bmc.2012.04.044. PMID 22607884.
- Voigt, Oliver; Adamski, Marcin; Sluzek, Kasia; Adamska, Maja (2014). "Calcareous sponge genomes reveal complex evolution of α-carbonic anhydrases and two key biomineralization enzymes". BMC Evolutionary Biology. 14: 230. doi:10.1186/s12862-014-0230-z. PMC 4265532. PMID 25421146.
- Jackson, D. J.; Macis, L.; Reitner, J.; Degnan, B. M.; Worheide, G. (2007). "Sponge Paleogenomics Reveals an Ancient Role for Carbonic Anhydrase in Skeletogenesis". Science. 316 (5833): 1893–1895. Bibcode:2007Sci...316.1893J. doi:10.1126/science.1141560. PMID 17540861. S2CID 7042860.
- Germer, Juliane; Mann, Karlheinz; Wörheide, Gert; Jackson, Daniel John (2015). "The Skeleton Forming Proteome of an Early Branching Metazoan: A Molecular Survey of the Biomineralization Components Employed by the Coralline Sponge Vaceletia Sp". PLOS ONE. 10 (11): e0140100. Bibcode:2015PLoSO..1040100G. doi:10.1371/journal.pone.0140100. PMC 4633127. PMID 26536128.
- Voigt, Oliver; Fradusco, Benedetta; Gut, Carolin; Kevrekidis, Charalampos; Vargas, Sergio; Wörheide, Gert (2021). "Carbonic Anhydrases: An Ancient Tool in Calcareous Sponge Biomineralization". Frontiers in Genetics. 12: 624533. doi:10.3389/fgene.2021.624533. PMC 8058475. PMID 33897759.
Material was copied from this source, which is available under a Creative Commons Attribution 4.0 International License.
- Manuel, M. (2006). "Phylogeny and evolution of calcareous sponges". Canadian Journal of Zoology. 84 (2): 225–241. doi:10.1139/z06-005.
- Voigt, Oliver; Adamska, Maja; Adamski, Marcin; Kittelmann, André; Wencker, Lukardis; Wörheide, Gert (2017). "Spicule formation in calcareous sponges: Coordinated expression of biomineralization genes and spicule-type specific genes". Scientific Reports. 7: 45658. Bibcode:2017NatSR...745658V. doi:10.1038/srep45658. PMC 5390275. PMID 28406140.
- Imsiecke G, Steffen R, Custodio M, Borojevic R, Müller WE (August 1995). "Formation of spicules by sclerocytes from the freshwater sponge Ephydatia muelleri in short-term cultures in vitro". In Vitro Cell Dev Biol Anim. 31 (7): 528–35. doi:10.1007/BF02634030. PMID 8528501. S2CID 22067910.
- Minchin, E. A. (1898). "Memoirs: Materials for a Monograph of the Ascons.--I. On the Origin and Growth of the Triradiate and Quadriradiate Spicules in the Family Clathrinidæ". Journal of Cell Science (160): 469–587. doi:10.1242/jcs.s2-40.160.469.
- Woodland, W. (1905). "Memoirs: Studies in Spicule Formation". Journal of Cell Science. 52 (194): 231–282. doi:10.1242/jcs.s2-49.194.231.
- Ledger, Philipw.; Jones, W.Clifford (1977). "Spicule formation in the calcareous sponge Sycon ciliatum". Cell and Tissue Research. 181 (4): 553–567. doi:10.1007/BF00221776. PMID 884721. S2CID 9916021.
- Ilan, Micha; Aizenberg, Joanna; Gilor, Osnat (1996). "Dynamics and growth patterns of calcareous sponge spicules". Proceedings of the Royal Society of London. Series B: Biological Sciences. 263 (1367): 133–139. Bibcode:1996RSPSB.263..133I. doi:10.1098/rspb.1996.0021. S2CID 85345222.
- Susanne Gatti (2002). "The Role of Sponges in High-Antarctic Carbon and Silicon Cycling - a Modelling Approach" (PDF). Ber. Polarforsch. Meeresforsch. 434. ISSN 1618-3193. Archived from the original (PDF) on 2011-07-24. Retrieved 2009-05-25.
- Van Soest, Rob W. M.; Boury-Esnault, Nicole; Vacelet, Jean; Dohrmann, Martin; Erpenbeck, Dirk; De Voogd, Nicole J.; Santodomingo, Nadiezhda; Vanhoorne, Bart; Kelly, Michelle; Hooper, John N. A. (2012-04-27). "Global Diversity of Sponges (Porifera)". PLOS ONE. Public Library of Science (PLoS). 7 (4): e35105. Bibcode:2012PLoSO...735105V. doi:10.1371/journal.pone.0035105. ISSN 1932-6203. PMC 3338747. PMID 22558119.
- Cárdenas, Paco (2020). "Surface Microornamentation of Demosponge Sterraster Spicules, Phylogenetic and Paleontological Implications". Frontiers in Marine Science. 7. doi:10.3389/fmars.2020.613610.
Material was copied from this source, which is available under a Creative Commons Attribution 4.0 International License.
- Donati, V. (1753). "Auszug seiner Natur-Geschichte des Adriatischen Meeres", Halle: Palala Press.
- Sollas, W. J. (1888). "Report on the Tetractinellida collected by H.M.S. Challenger, during the years 1873-1876". Report on the Scientific Results of the Voyage of H.M.S. Challenger, 1873-1876. Zoology, 25: 1–458.
- Hanitsch, R. (1895). "Notes on a collection of sponges from the West Coast of Portugal". Trans. Liverpool Biol. Soc., 9: 205–219.
- von Lendenfeld, R. (1910). "The Sponges. 2. The erylidae". In: Reports on the scientific results of the expedition to the eastern tropical pacific, in charge of Alexander Agassiz, by the U.S. Fish Commission Steamer 'Albatross', from October, 1904, to March, 1905, Lieut. Commander L.M. Garrett, U.S.N., Commanding, and of other Expeditions of the Albatross, 1888-1904. (21). Mem. Museum Comp. Zool., 41: 261–324.
- Cã¡Rdenas, Paco; Rapp, Hans Tore; Schander, Christoffer; Tendal, Ole S. (2010). "Molecular taxonomy and phylogeny of the Geodiidae (Porifera,Demospongiae, Astrophorida) – combining phylogenetic and Linnaean classification". Zoologica Scripta. 39: 89–106. doi:10.1111/j.1463-6409.2009.00402.x. hdl:1956/4020. S2CID 85137461.
- Cárdenas, Paco; Xavier, Joana R.; Reveillaud, Julie; Schander, Christoffer; Rapp, Hans Tore (2011). "Molecular Phylogeny of the Astrophorida (Porifera, Demospongiaep) Reveals an Unexpected High Level of Spicule Homoplasy". PLOS ONE. 6 (4): e18318. Bibcode:2011PLoSO...618318C. doi:10.1371/journal.pone.0018318. PMC 3072971. PMID 21494664.
- Santucci, R. (1922). "La Geodia cydonium come centro di associazione biologica". Mem. Comitato Talassografico ltaliano: 103: 5–19.
- Corriero, G. (1987) (1989). "The sponge fauna from the Stagnone di Marsala (Sicily): taxonomic and ecological observations". Bollettino dei musei e degli istituti biologici dell, Univ. Genova, 53: 101–113.
- Cárdenas, Paco; Rapp, Hans Tore; Klitgaard, Anne Birgitte; Best, Megan; Thollesson, Mikael; Tendal, Ole Secher (2013). "Taxonomy, biogeography and DNA barcodes of Geodiaspecies (Porifera, Demospongiae, Tetractinellida) in the Atlantic boreo-arctic region". Zoological Journal of the Linnean Society. 169 (2): 251–311. doi:10.1111/zoj.12056. S2CID 85922551.
- Becking, Leontine (2013). "Revision of the genus Placospongia (Porifera, Demospongiae, Hadromerida, Placospongiidae) in the Indo-West Pacific". ZooKeys (298): 39–76. doi:10.3897/zookeys.298.1913. PMC 3689107. PMID 23794884.
- Hajdu, E., Peixinho, S., and Fernandez, J. C. C. (2011). "Esponjas Marinhas da Bahia - Guia de Campo e Laboratório". Série Livros, 45. Rio de Janeiro: Museu Nacional/UFRJ.
- Cárdenas, Paco; Menegola, Carla; Rapp, Hans Tore; Díaz, Maria Cristina (2009). "Morphological description and DNA barcodes of shallow-water Tetractinellida (Porifera: Demospongiae) from Bocas del Toro, Panama, with description of a new species". Zootaxa. 2276: 1–39. doi:10.11646/zootaxa.2276.1.1.
- Cárdenas, Paco; Rapp, Hans Tore (2013). "Disrupted spiculogenesis in deep-water Geodiidae (Porifera, Demospongiae) growing in shallow waters". Invertebrate Biology. 132 (3): 173–194. doi:10.1111/ivb.12027.
- Cárdenas, Paco; Vacelet, Jean; Chevaldonné, Pierre; Pérez, Thierry; Xavier, Joana R. (2018). "From marine caves to the deep sea, a new look at Caminella (Demospongiae, Geodiidae) in the Atlanto-Mediterranean region". Zootaxa. 4466 (1): 174–196. doi:10.11646/zootaxa.4466.1.14. PMID 30313446. S2CID 52976107.
- Krasko, Anatoli; Lorenz, Bernd; Batel, Renato; Schröder, Heinz C.; Müller, Isabel M.; Müller, Werner E. G. (2000). "Expression of silicatein and collagen genes in the marine sponge Suberites domuncula is controlled by silicate and myotrophin". European Journal of Biochemistry. 267 (15): 4878–4887. doi:10.1046/j.1432-1327.2000.01547.x. PMID 10903523.
- Custódio, Márcio; Hajdu, Eduardo; Muricy, Guilherme (2002). "In vivo study of microsclere formation in sponges of the genus Mycale (Demospongiae, Poecilosclerida)". Zoomorphology. 121 (4): 203–211. doi:10.1007/s00435-002-0057-9. S2CID 39893025.
- Müller, Werner E. G.; Rothenberger, Matthias; Boreiko, Alexandra; Tremel, Wolfgang; Reiber, Andreas; Schröder, Heinz C. (2005). "Formation of siliceous spicules in the marine demosponge Suberites domuncula". Cell and Tissue Research. 321 (2): 285–297. doi:10.1007/s00441-005-1141-5. PMID 15947968. S2CID 12720013.
- Shimizu, K.; Cha, J.; Stucky, G. D.; Morse, D. E. (1998). "Silicatein : Cathepsin L-like protein in sponge biosilica". Proceedings of the National Academy of Sciences. 95 (11): 6234–6238. doi:10.1073/pnas.95.11.6234. PMC 27641. PMID 9600948.
- Simpson, Tracy L.; Langenbruch, Paul-Friedrich; Scalera-Liaci, Lidia (1985). "Silica spicules and axial filaments of the marine sponge Stelletta grubii (Porifera, Demospongiae)". Zoomorphology. 105 (6): 375–382. doi:10.1007/BF00312281. S2CID 35458775.
- Uriz, María J.; Turon, Xavier; Becerro, Mikel A. (2000). "Silica deposition in Demosponges: Spiculogenesis in Crambe crambe". Cell and Tissue Research. 301 (2): 299–309. doi:10.1007/s004410000234. PMID 10955725. S2CID 21428171.
- Uriz, María-J. (2006). "Mineral skeletogenesis in sponges". Canadian Journal of Zoology. 84 (2): 322–356. doi:10.1139/z06-032.
- Pisera, Andrzej (2003). "Some aspects of silica deposition in lithistid demosponge desmas". Microscopy Research and Technique. 62 (4): 312–326. doi:10.1002/jemt.10398. PMID 14534905. S2CID 26495972.
- Simpson, T. L. (6 December 2012). The Cell Biology of Sponges. Springer. ISBN 9781461252146.
- Simpson, Tracy L. (1989). "Silicification Processes in Sponges: Geodia Asters and the Problem of Morphogenesis of Spicule Shape". Origin, Evolution, and Modern Aspects of Biomineralization in Plants and Animals. pp. 125–136. doi:10.1007/978-1-4757-6114-6_9. ISBN 978-1-4757-6116-0.
- Müller, Werner E.G.; Wang, Xiaohong; Burghard, Zaklina; Bill, Joachim; Krasko, Anatoli; Boreiko, Alexandra; Schloßmacher, Ute; Schröder, Heinz C.; Wiens, Matthias (2009). "Bio-sintering processes in hexactinellid sponges: Fusion of bio-silica in giant basal spicules from Monorhaphis chuni☆". Journal of Structural Biology. 168 (3): 548–561. doi:10.1016/j.jsb.2009.08.003. PMID 19683578.
- Werner, Peter; Blumtritt, Horst; Zlotnikov, Igor; Graff, Andreas; Dauphin, Yannicke; Fratzl, Peter (2015). "Electron microscope analyses of the bio-silica basal spicule from the Monorhaphis chuni sponge". Journal of Structural Biology. 191 (2): 165–174. doi:10.1016/j.jsb.2015.06.018. PMID 26094876.
- Reiswig, Henry M. (2002). "Class Hexactinellida Schmidt, 1870". Systema Porifera. pp. 1201–1210. doi:10.1007/978-1-4615-0747-5_123. ISBN 978-0-306-47260-2.
- Rützler, K.; MacIntyre, I. G. (1978). "Siliceous sponge spicules in coral reef sediments". Marine Biology. 49 (2): 147–159. doi:10.1007/BF00387114. S2CID 86814441.
- Harrison, Frederick W.; Warner, Barry G. (1986). "Fossil Fresh-Water Sponges (Porifera: Spongillidae) from Western Canada: An Overlooked Group of Quaternary Paleoecological Indicators". Transactions of the American Microscopical Society. 105 (2): 110–120. doi:10.2307/3226383. JSTOR 3226383.
- Gutt, J.; Böhmer, A.; Dimmler, W. (2013). "Antarctic sponge spicule mats shape macrobenthic diversity and act as a silicon trap". Marine Ecology Progress Series. 480: 57–71. Bibcode:2013MEPS..480...57G. doi:10.3354/meps10226.
- Koltun, V.M. (1968). "Spicules of sponges as an element of the bottom sediments of the Antarctic". In: SCAR Symposium on Antarctic Oceanography, Scott Polar Research Institute, pages 21–123.
- Gutt, Julian (2007). "Antarctic macro-zoobenthic communities: A review and an ecological classification". Antarctic Science. 19 (2): 165–182. Bibcode:2007AntSc..19..165G. doi:10.1017/S0954102007000247. S2CID 129803464.
- Cayeux L. (1929). "Les roches sédimentaires de France: roches siliceuses". Mémoires de la Carte Géologique de France 1929, pages 1-774
- Gammon, P.R., Middleton, G.V., Church, M.J., Coniglio, M., Hardie, L.A. and Longstaffe, F.J. (1978) "Spiculites and spongolites}. In: Encyclopedia of Sediments and Sedimentary Rocks, Dordrecht: Springer.
- Reid, C.M.; James, N.P.; Kyser, T.K.; Barrett, N.; Hirst, A.J. (2008). "Modern estuarine siliceous spiculites, Tasmania, Australia: A non-polar link to Phanerozoic spiculitic cherts". Geology. 36 (2): 107. Bibcode:2008Geo....36..107R. doi:10.1130/G24333A.1.
- Schindler, Thomas; Wuttke, Michael; Poschmann, Markus (2008). "Oldest record of freshwater sponges (Porifera: Spongillina) — spiculite finds in the Permo-Carboniferous of Europe". Paläontologische Zeitschrift. 82 (4): 373–384. doi:10.1007/BF03184428. S2CID 129770411.
- Gates, Laura M.; James, Noel P.; Beauchamp, Benoit (2004). "A glass ramp: Shallow-water Permian spiculitic chert sedimentation, Sverdrup Basin, Arctic Canada". Sedimentary Geology. 168 (1–2): 125–147. Bibcode:2004SedG..168..125G. doi:10.1016/j.sedgeo.2004.03.008.
- Ritterbush, Kathleen (2019). "Sponge meadows and glass ramps: State shifts and regime change". Palaeogeography, Palaeoclimatology, Palaeoecology. 513: 116–131. Bibcode:2019PPP...513..116R. doi:10.1016/j.palaeo.2018.08.009. S2CID 134003011.
- Morganti, Teresa M. (2021). "In situ observation of sponge trails suggests common sponge locomotion in the deep central Arctic". Current Biology. 31 (8): R368–R370. doi:10.1016/j.cub.2021.03.014. PMID 33905688. S2CID 233429396.
- Riisgård, Hans Ulrik; Larsen, Poul S. (1995). "Filter-Feeding in Marine Macro-Invertebrates: Pump Characteristics, Modelling and Energy Cost". Biological Reviews. 70 (1): 67–106. doi:10.1111/j.1469-185X.1995.tb01440.x. PMID 21545387. S2CID 28635129.
- Muricy, Guilherme; Díaz, Maria Cristina (2002). "Order Homosclerophorida Dendy, 1905, Family Plakinidae Schulze, 1880". Systema Porifera. pp. 71–82. doi:10.1007/978-1-4615-0747-5_6. ISBN 978-0-306-47260-2.
- Manuel, Michaël; Borojevic, Radovan; Boury-Esnault, Nicole; Vacelet, Jean (2002). "Class Calcarea Bowerbank, 1864". Systema Porifera. pp. 1103–1116. doi:10.1007/978-1-4615-0747-5_115. ISBN 978-0-306-47260-2.
- Lukowiak, M.; Pisera, A.; O'Dea, A. (2013). "Do Spicules in Sediments Reflect the Living Sponge Community? A Test in a Caribbean Shallow-Water Lagoon". PALAIOS. 28 (6): 373–385. Bibcode:2013Palai..28..373L. doi:10.2110/palo.2012.p12-082r. S2CID 2072201.
- Łukowiak, Magdalena (2016). "Spicular analysis of surficial sediments as a supplementary tool for studies of modern sponge communities". Helgoland Marine Research. 70. doi:10.1186/s10152-016-0459-6. S2CID 9536558.
- Volkmer-Ribeiro, C.; Reitner, J. (1991). "Renewed Study of the Type Material of Palaeospongilla chubutensis Ott and Volkheimer (1972)". Fossil and Recent Sponges. pp. 121–133. doi:10.1007/978-3-642-75656-6_10. hdl:11858/00-1735-0000-0001-33B1-2. ISBN 978-3-642-75658-0.
- Olszewska-Nejbert, Danuta; Świerczewska-Gładysz, Ewa (2013). "Cenomanian (Late Cretaceous) siliceous sponges from Nezvys'ko and Rakovets' (Pokuttian Podillia, western Ukraine)". Cretaceous Research. 43: 116–144. doi:10.1016/j.cretres.2013.03.001.
- Łukowiak, M.; Pisera, A. (2017). "Bodily preserved Eocene non-lithistid demosponge fauna from southern Australia: Taxonomy and affinities". Journal of Systematic Palaeontology. 15 (6): 473–497. doi:10.1080/14772019.2016.1197329. S2CID 89387705.
- Diaz, M.C. and Rützler, K. (2001). "Sponges: an essential component of Caribbean coral reefs". Bulletin of marine science, 69(2): 535–546.
- Collin, R., Diaz, M.C., Norenburg, J.L., Rocha, R.D., Sanchez, J.A., Schulz, A., Schwartz, M.L. and Valdes, A. (2005) "Photographic identification guide to some common marine invertebrates of Bocas Del Toro, Panama". Caribbean Journal of Science.
- Pisera, A. (2006). "Palaeontology of sponges — a review". Canadian Journal of Zoology. 84 (2): 242–261. doi:10.1139/z05-169.
- Aizenburg, Joanna; et al. (2004). "Biological glass fibers: Correlation between optical and structural properties". Proceedings of the National Academy of Sciences. 101 (10): 3358–3363. Bibcode:2004PNAS..101.3358A. doi:10.1073/pnas.0307843101. PMC 373466. PMID 14993612.
- Aizenberg, J.; Sundar, V. C.; Yablon, A. D.; Weaver, J. C.; Chen, G. (March 2004). "Biological glass fibers: Correlation between optical and structural properties". Proceedings of the National Academy of Sciences. 101 (10): 3358–3363. Bibcode:2004PNAS..101.3358A. doi:10.1073/pnas.0307843101. PMC 373466. PMID 14993612.
- Brümmer, Franz; Pfannkuchen, Martin; Baltz, Alexander; Hauser, Thomas; Thiel, Vera (2008). "Light inside sponges". Journal of Experimental Marine Biology and Ecology. 367 (2): 61–64. doi:10.1016/j.jembe.2008.06.036.
- "Nature's 'fibre optics' experts". BBC. 2008-11-10. Retrieved 2008-11-10.
Further references
- Shoham, Erez; Prohaska, Thomas; Barkay, Zahava; Zitek, Andreas; Benayahu, Yehuda (2019). "Soft corals form aragonite-precipitated columnar spiculite in mesophotic reefs". Scientific Reports. 9 (1): 1241. Bibcode:2019NatSR...9.1241S. doi:10.1038/s41598-018-37696-z. PMC 6362064. PMID 30718658.