Bispecific monoclonal antibody
A bispecific monoclonal antibody (BsMAb, BsAb) is an artificial protein that can simultaneously bind to two different types of antigen or two different epitopes on the same antigen.[1] Naturally occurring antibodies typically only target one antigen. BsAbs can be manufactured in several structural formats. BsAbs can be designed to recruit and activate immune cells, to interfere with receptor signaling and inactivate signaling ligands, and to force association of protein complexes.[2] BsAbs have been explored for cancer immunotherapy, drug delivery, and Alzeimer's disease.[1][3]
Development history
The original concept of BsAbs was proposed by Nisonoff and his collaborators in the 1960s, including the first idea of antibody architecture and other findings.[4][5] In 1975, the problem of producing pure antibodies was solved by the creation of hybridoma technology, and the new era of monoclonal antibodies (MoAbs) came.[6] In 1983, Milstein and Cuello created hybrid-hybridoma (quadroma) technology.[7] In 1988, the single-chain variable fragment (scFv) was invented by the Huston team to minimize the refolding problems, which contains the incorrect domain pairing or aggregation of two-chain species.[8] In 1996, the BsAbs became more developed when the knobs-into-holes technology emerged.[1][9]
Structural types and manufacturing methods
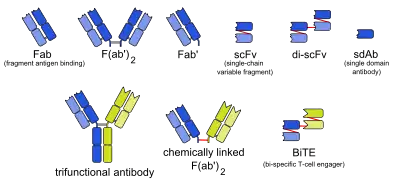
There are many formats of BsAbs, but the two main categories are IgG-like and non-IgG-like.[3] The main types of manufacturing methods are quadromas, chemical conjugation, and genetic recombination, and each method results in a unique format.[3][10]
IgG-like
This format retains the traditional monoclonal antibody (mAb) structure of two Fab arms and one Fc region, except the two Fab sites bind different antigens. The most common types are called trifunctional antibodies, as they have three unique binding sites on the antibody: the two Fab regions, and the Fc region. Each heavy and light chain pair is from a unique mAb. The Fc region made from the two heavy chains forms the third binding site. These BsAbs are often manufactured with the quadroma, or the hybrid hybridoma, method.[11][12][13]
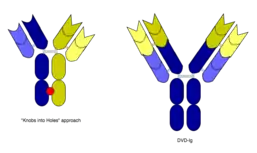
However, the quadroma method relies on random chance to form usable BsAb, and can be inefficient. Another method for manufacturing IgG-like BsAb is called "knobs into holes," and relies on introducing a mutation for a large amino acid in the heavy chain from one mAb, and a mutation for a small amino acid in the other mAb's heavy chain. This allows the target heavy chains (and their corresponding light chains) to fit together better, and makes the production of BsAbs more reliable.[3][10]
Non-IgG-like
There are other BsAbs that lack an Fc region entirely, and thus leads to relatively simple design strategies.[1] These include chemically linked Fabs, consisting of only the Fab regions, and various types of bivalent and trivalent single-chain variable fragments (ScFvs). There are also fusion proteins mimicking the variable domains of two antibodies. The furthest developed of these newer formats are the bi-specific T-cell engagers (BiTEs), which uses the G4S linker to connect two ScFvs-one CD3 antibody ScFv and one tumor-associated anntigen (TAA) or tumor-specific ScFv-to redirect T cells to cancer cells for target killing.[14][15][16] Other platforms include tetravalent antiparallel structure (TandAbs) and VH only (Bi-Nanobody). The TandAb platform is formed by a tetravalent antibody molecule containing two binding sites for each of two antigens.[17] In this platform, the reverse pairing of two peptide chains forms a homodimer molecule. As an example, AFM11 is based on the TandAbs platform and targets both CD3 and CD19 to achieve therapeutic effects. AFM11 showed dose-dependent inhibition of Raji tumors in vivo.[18] The Bi-Nanobody platform forms multi-specific binding through the connection between the VH regions of two or more antibody molecules. The products that are designed based on this platform are small molecules and these small molecules have high stability and better tissue permeability in vivo.[19] Even though non-IgG-like BsAbs have low molecular weight and thus high tumor tissue permeability, their half-life is relatively short and they require multiple doses.[1]
Mechanism of action
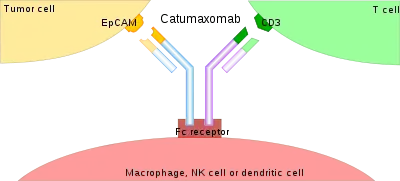
Recruiting and activating of immune cells
The binding of a BsAb to its target antigens can lead to a variety of effects. The most widely used application of this approach is in cancer immunotherapy, where BsAbs are engineered to simultaneously bind a cytotoxic cell and a target (a tumour cell) to be destroyed. It is possible to observe the bridging effect that BsAbs have on T cell/cancer cell interactions using label-free live cell imaging. Catumaxomab, one of the first trifunctional antibodies approved for therapeutic use, binds both CD3 on cytotoxic T cells and EpCAM on human adenocarcinomas.[11][12] The Fc region additionally binds to a cell that expresses Fc receptors, like a macrophage, natural killer cell or dendritic cell. Since the Fc region is still intact, this allows for the BsAb to trigger common immune responses when recognized by an Fc receptor, such as antibody-dependent cell-mediated cytotoxicity or complement-dependent cytotoxicity.[13][15]
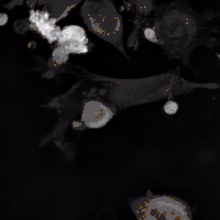
Interfering with receptor signaling and inactivating signaling ligands
The growth of tumor cells can be simulated or modulated by receptor tyrosine kinase (RTKs), including members of the Her family or insulin-like growth factor (IGF). The RTKs are therefore preferred targets in cancer therapy. Although monospecific RTK-targeting IgGs have already been available in the market, such as cetuximab (ErbituxTM) and panitumumab (VectibixTM), both of which are directed against HER1. However, cancer cells can switch to a different pathway to escape the growth inhibition generated by blocking one signaling pathway. To improve the therapeutic efficacy, simultaneously interfering/blocking of two (or more) RTK signaling pathways, achieved through the mediation of BsAb to inactivate either the RTKs or their ligand, reduces the possibility of the escape mechanisms adopted by the tumor cells.[20][21]
In addition, in working with Ebolavirus vaccines, a study has shown that a DVD-Ig antibody can be used to prevent viral escape from the endosome. Ebolaviruses infect cells by receptor-mediated endocytosis. Researchers developed DVD-Igs where the outer variable regions bind to the surface glycoproteins of the viral coat and enter the cell with the virus. These outer regions are cleaved in the viral endosome, revealing the inner variable regions that then bind to both the virus and internal receptors in the endosome. Blocking the interaction between the virus and endosomal proteins prevents viral escape from the endosome and further infection.[22]
Forcing association of protein complexes
As an example, RG6013 (Chugai) is an IgG derivative containing H-chain heterodimerization motifs, which was combined with the common light chain approach to prevent L-chain mispairing issues.[23][24] With a bivalent composition, RG6013 brings two protein antigens together into one complex. Factor IXa and Factor X in the coagulation cascade are the cognate antigens which are bound by RG6013. These two factors are brought together by coagulation factor VIIIa in a healthy individual, while patients with bleeding disorder hemophilia A do not have VIIIa. Current treatment of this disorder is to supplement the patients with FVIII to reduce bleeding complications. But FVIII can be recognized as a foreign protein in these patients due to the absence of this protein and thus an immune response will be generated against this protein. Besides, FVIII has a short half-life (less than 15 hours) and thus is cleared rapidly. However, the humanized BsAb has lower immunogenicity and long serum half-life compared with FVIII and thus provide a better treatment for hemophilia.[2]
Advantages over ordinary monoclonal antibodies
Cancer immunotherapy with ordinary monoclonal antibodies does not activate T-lymphocytes because the Fab regions are already used for binding the tumor cells, and this type of cell does not have Fc receptors.[25] Bispecific antibodies also have a higher cytotoxic potential, and bind to antigens that are expressed relatively weakly.[26] The effective dose is around 0.01 mg·m−2·d−1 (milligrams per square meter body surface area per day), which is several orders of magnitude lower than with ordinary antibodies.[25] For non-IgG-like BsAbs, their smaller size allows them to reach antigens usually unavailable to conventional antibodies.[3] In the case of Ebola vaccines, this method allows the antibody to target intracellular targets not usually accessible by traditional monoclonal antibody treatments.[22]
Additionally, targeting more than one molecule can be useful to circumvent the regulation of parallel pathways and avoid resistance to the treatment. Binding or blocking multiple targets in a pathway can be beneficial to stopping disease, as most conditions have complicated multifaceted effects throughout the body.[27] Together with combination therapies, BsAbs are being used more and more to treat certain types of cancers, as, over time, some tumors develop resistances to checkpoint inhibitors and/or co-stimulatory molecules.[28]
Problems and current disadvantages
IgG-like antibodies can be immunogenic, which means the Fc region could cause detrimental downstream immune responses caused by cells that are activated by Fc receptors.[3] The therapeutic use of BsAbs as a whole is still largely in development, with many clinical trials currently ongoing that are determining the efficacy and safety of BsAbs for treatment.[14]
Applications
Bispecific antibodies have a wide variety of applications in diagnosis and therapy. BsAbs can be combined with HRPO, can be used in pre-targeting strategies, and can be used to provide better imaging for early detection in diagnosis. To treat cancer, BsAbs can target immune cells precisely, help and reactive the immune cells, fine-tune the fate and function of immune cells, improve the tolerance of immune cells, and promote the return to immune homeostasis. BsAbs can also be applied to treat other diseases, including hemophilia A, diabetes, Alzeimer's disease, and ophthalmological diseases.[1]
BsAbs on the market
Three bispecific antibodies are presently in clinical use. Blinatumomab, which targets CD19 and CD3, is used in the treatment of Philadelphia chromosome negative B cell acute lymphoblastic leukemia (ALL). Emicizumab, which targets clotting factors IXa and X, is used in the treatment of hemophilia A.[29] Catumaxomab was withdrawn from the European market in 2017 for commercial reasons.[30] Amivantamab, which targets epidermal growth factor (EGF) and MET receptors, for adult patients with locally advanced or metastatic non-small cell lung cancer (NSCLC) with epidermal growth factor receptor (EGFR) exon 20 insertion mutations.[31]
References
This article incorporates public domain material from Dictionary of Cancer Terms. U.S. National Cancer Institute.
- Ma J, Mo Y, Tang M, Shen J, Qi Y, Zhao W, et al. (2021). "Bispecific Antibodies: From Research to Clinical Application". Frontiers in Immunology. 12: 626616. doi:10.3389/fimmu.2021.626616. PMC 8131538. PMID 34025638.
- Kontermann RE, Brinkmann U (July 2015). "Bispecific antibodies". Drug Discovery Today. 20 (7): 838–847. doi:10.1016/j.drudis.2015.02.008. PMID 25728220.
- Fan G, Wang Z, Hao M, Li J (December 2015). "Bispecific antibodies and their applications". Journal of Hematology & Oncology. 8: 130. doi:10.1186/s13045-015-0227-0. PMC 4687327. PMID 26692321.
- Nisonoff A, Wissler FC, Lipman LN (December 1960). "Properties of the major component of a peptic digest of rabbit antibody". Science. 132 (3441): 1770–1771. Bibcode:1960Sci...132.1770N. doi:10.1126/science.132.3441.1770. PMID 13729245. S2CID 19267292.(subscription required)
- Nisonoff A, Rivers MM (May 1961). "Recombination of a mixture of univalent antibody fragments of different specificity". Archives of Biochemistry and Biophysics. 93 (2): 460–462. doi:10.1016/0003-9861(61)90296-x. PMID 13729244.(subscription required)
- Köhler G, Milstein C (August 1975). "Continuous cultures of fused cells secreting antibody of predefined specificity". Nature. 256 (5517): 495–497. Bibcode:1975Natur.256..495K. doi:10.1038/256495a0. PMID 1172191. S2CID 4161444.(subscription required)
- Milstein C, Cuello AC (October 1983). "Hybrid hybridomas and their use in immunohistochemistry". Nature. 305 (5934): 537–540. Bibcode:1983Natur.305..537M. doi:10.1038/305537a0. PMID 6137772. S2CID 4264730.(subscription required)
- Huston JS, Levinson D, Mudgett-Hunter M, Tai MS, Novotný J, Margolies MN, et al. (August 1988). "Protein engineering of antibody binding sites: recovery of specific activity in an anti-digoxin single-chain Fv analogue produced in Escherichia coli". Proceedings of the National Academy of Sciences of the United States of America. 85 (16): 5879–5883. Bibcode:1988PNAS...85.5879H. doi:10.1073/pnas.85.16.5879. PMC 281868. PMID 3045807.
- Merchant AM, Zhu Z, Yuan JQ, Goddard A, Adams CW, Presta LG, Carter P (July 1998). "An efficient route to human bispecific IgG". Nature Biotechnology. 16 (7): 677–681. doi:10.1038/nbt0798-677. PMID 9661204. S2CID 41622760.(subscription required)
- Liu H, Saxena A, Sidhu SS, Wu D (2017-01-01). "Fc Engineering for Developing Therapeutic Bispecific Antibodies and Novel Scaffolds". Frontiers in Immunology. 8: 38. doi:10.3389/fimmu.2017.00038. PMC 5266686. PMID 28184223.
- Müller D, Kontermann RE (April 2010). "Bispecific antibodies for cancer immunotherapy: Current perspectives". BioDrugs. 24 (2): 89–98. doi:10.2165/11530960-000000000-00000. PMID 20199124. S2CID 27797190.
- Chames P, Baty D (2009). "Bispecific antibodies for cancer therapy: the light at the end of the tunnel?". mAbs. 1 (6): 539–547. doi:10.4161/mabs.1.6.10015. PMC 2791310. PMID 20073127.
- Lindhofer H, Mocikat R, Steipe B, Thierfelder S (July 1995). "Preferential species-restricted heavy/light chain pairing in rat/mouse quadromas. Implications for a single-step purification of bispecific antibodies". Journal of Immunology. 155 (1): 219–225. PMID 7602098.
- Yang F, Wen W, Qin W (December 2016). "Bispecific Antibodies as a Development Platform for New Concepts and Treatment Strategies". International Journal of Molecular Sciences. 18 (1): 48. doi:10.3390/ijms18010048. PMC 5297683. PMID 28036020.
- Baeuerle PA, Reinhardt C (June 2009). "Bispecific T-cell engaging antibodies for cancer therapy". Cancer Research. 69 (12): 4941–4944. doi:10.1158/0008-5472.CAN-09-0547. PMID 19509221.
- Wozniak-Knopp G, Bartl S, Bauer A, Mostageer M, Woisetschläger M, Antes B, et al. (April 2010). "Introducing antigen-binding sites in structural loops of immunoglobulin constant domains: Fc fragments with engineered HER2/neu-binding sites and antibody properties". Protein Engineering, Design & Selection. 23 (4): 289–297. doi:10.1093/protein/gzq005. PMID 20150180.
- Kipriyanov SM, Moldenhauer G, Schuhmacher J, Cochlovius B, Von der Lieth CW, Matys ER, Little M (October 1999). "Bispecific tandem diabody for tumor therapy with improved antigen binding and pharmacokinetics". Journal of Molecular Biology. 293 (1): 41–56. doi:10.1006/jmbi.1999.3156. PMID 10512714.
- Reusch U, Duell J, Ellwanger K, Herbrecht C, Knackmuss SH, Fucek I, et al. (2015-05-04). "A tetravalent bispecific TandAb (CD19/CD3), AFM11, efficiently recruits T cells for the potent lysis of CD19(+) tumor cells". mAbs. 7 (3): 584–604. doi:10.1080/19420862.2015.1029216. PMC 4622993. PMID 25875246.
- Bannas P, Hambach J, Koch-Nolte F (2017). "Nanobodies and Nanobody-Based Human Heavy Chain Antibodies As Antitumor Therapeutics". Frontiers in Immunology. 8: 1603. doi:10.3389/fimmu.2017.01603. PMC 5702627. PMID 29213270.
- Yarden Y, Sliwkowski MX (February 2001). "Untangling the ErbB signalling network". Nature Reviews. Molecular Cell Biology. 2 (2): 127–137. doi:10.1038/35052073. PMID 11252954. S2CID 4235503.
- Slamon DJ, Clark GM, Wong SG, Levin WJ, Ullrich A, McGuire WL (January 1987). "Human breast cancer: correlation of relapse and survival with amplification of the HER-2/neu oncogene". Science. 235 (4785): 177–182. Bibcode:1987Sci...235..177S. doi:10.1126/science.3798106. PMID 3798106.
- Wec AZ, Nyakatura EK, Herbert AS, Howell KA, Holtsberg FW, Bakken RR, et al. (October 2016). "A "Trojan horse" bispecific-antibody strategy for broad protection against ebolaviruses". Science. 354 (6310): 350–354. Bibcode:2016Sci...354..350W. doi:10.1126/science.aag3267. PMC 5647781. PMID 27608667.
- Kitazawa T, Igawa T, Sampei Z, Muto A, Kojima T, Soeda T, et al. (October 2012). "A bispecific antibody to factors IXa and X restores factor VIII hemostatic activity in a hemophilia A model". Nature Medicine. 18 (10): 1570–1574. doi:10.1038/nm.2942. PMID 23023498. S2CID 13125020.
- Sampei Z, Igawa T, Soeda T, Okuyama-Nishida Y, Moriyama C, Wakabayashi T, et al. (2013-02-28). "Identification and multidimensional optimization of an asymmetric bispecific IgG antibody mimicking the function of factor VIII cofactor activity". PLOS ONE. 8 (2): e57479. Bibcode:2013PLoSO...857479S. doi:10.1371/journal.pone.0057479. PMC 3585358. PMID 23468998.
- Bargou R, Leo E, Zugmaier G, Klinger M, Goebeler M, Knop S, et al. (August 2008). "Tumor regression in cancer patients by very low doses of a T cell-engaging antibody". Science. 321 (5891): 974–977. Bibcode:2008Sci...321..974B. doi:10.1126/science.1158545. PMID 18703743. S2CID 22628198.
- Weiner LM, Holmes M, Richeson A, Godwin A, Adams GP, Hsieh-Ma ST, et al. (September 1993). "Binding and cytotoxicity characteristics of the bispecific murine monoclonal antibody 2B1". Journal of Immunology. 151 (5): 2877–2886. PMID 8103070.
- Varela MA (July 2015). "Identification of sequences common to more than one therapeutic target to treat complex diseases: simulating the high variance in sequence interactivity evolved to modulate robust phenotypes". BMC Genomics. 16: 530. doi:10.1186/s12864-015-1727-6. PMC 4506634. PMID 26187740.
- Koustas E, Sarantis P, Papavassiliou AG, Karamouzis MV (April 2020). "The Resistance Mechanisms of Checkpoint Inhibitors in Solid Tumors". Biomolecules. 10 (5): 66–82. doi:10.3390/biom10050666. PMC 7277892. PMID 32344837.
- Suurs FV, Lub-de Hooge MN, de Vries EG, de Groot DJ (September 2019). "A review of bispecific antibodies and antibody constructs in oncology and clinical challenges". Pharmacology & Therapeutics. 201: 103–119. doi:10.1016/j.pharmthera.2019.04.006. PMID 31028837.
- "Removab: Withdrawal of the marketing authorisation in the European Union" (PDF). European Medicines Agency. 2017-07-10.
- "Amivantamab OK'd for EGFR-Mutant NSCLC". Cancer Discovery. 11 (7): 1604.1–1604. July 2021. doi:10.1158/2159-8290.CD-NB2021-0351. PMID 34083225. S2CID 235334950.
External links
- Bispecific monoclonal antibody entry in the public domain NCI Dictionary of Cancer Terms
- Bispecific+antibodies at the US National Library of Medicine Medical Subject Headings (MeSH)