Effects of climate change
The effects of climate change span the impacts on physical environment, ecosystems and human societies due to human-caused climate change. The future impact of climate change depends on how much nations reduce greenhouse gas emissions and adapt to climate change.[5][6] Effects that scientists predicted in the past—loss of sea ice, accelerated sea level rise and longer, more intense heat waves—are now occurring.[7] The changes in climate are not uniform across the Earth. In particular, most land areas have warmed faster than most ocean areas, and the Arctic is warming faster than most other regions.[8] The regional changes vary: at high latitudes it is the average temperature that is increasing, while for the oceans and tropics it is in particular the rainfall and the water cycle where changes are observed.[9] Global warming changes regional climate via the melting of ice, changes in the hydrological cycle (such as rainfall) and changing currents in the oceans.
Physical changes include extreme weather, glacier retreat, sea level rise, declines in Arctic sea ice, and changes in the timing of seasonal events (such as earlier spring flowering). Since 1970, the ocean has absorbed more than 90% of the excess heat in the climate system. Even if global surface temperature is stabilized, sea levels will continue to rise and the ocean will continue to absorb excess heat from the atmosphere for many centuries.[3]: 20 The uptake of carbon dioxide from the atmosphere is leading to ocean acidification.[3]: 9
Climate change has degraded land by raising temperatures, drying soils and increasing wildfire risk.[10] Recent warming has strongly affected natural biological systems.[11] Species worldwide are migrating poleward to colder areas. On land, may species move to higher elevations, whereas marine species find colder water at greater depths.[12] Between 1% and 50% of species on land were assessed to be at substantially higher risk of extinction due to climate change.[13] Coral reefs and shellfish are vulnerable to the combined threat of ocean warming and acidification.[14]
Food security and access to fresh water are at risk due to rising temperatures. Climate change has profound impacts on human health, directly via heat stress and indirectly via the spread of infectious diseases. The vulnerability and exposure of humans to climate change varies by economic sector and by country. Wealthy industrialised countries, which have emitted the most CO2, have more resources and so are the least vulnerable to global warming.[15] Economic sectors that are likely to be affected include agriculture, fisheries, forestry, energy, insurance, financial services, tourism, and recreation.[16] Some groups may be particularly at risk from climate change, such as the poor, women, children and indigenous peoples.[17][18] They also have much lower levels of capacity available for coping with environmental change. Climate change can result in environmental migration, especially in developing countries where people are directly dependent on land for food.[19][20]
Observed and future changes in temperature

Global warming affects all elements of Earth's climate system.[21] Global surface temperatures have risen by 1 °C and are expected to rise further in the future.[21][22] Night-time temperatures have increased faster than daytime temperatures.[23] The impact on the environment, wildlife, society and humanity depends on how much more the Earth warms.[24]
One of the methods scientists use to predict the effects of human-caused climate change is to investigate past natural changes in climate.[25] To assess changes in Earth's past climate scientists have studied tree rings, ice cores, corals, and ocean and lake sediments.[26] These show that recent warming has surpassed anything in the last 2,000 years.[27] By the end of the 21st century, temperatures may increase to a level not experienced since the mid-Pliocene, around 3 million years ago.[28] At that time, mean global temperatures were about 2–4 °C warmer than pre-industrial temperatures, and the global mean sea level was up to 25 meters higher than it is today.[29]
How much the world warms depends on what humans do or not to limit GHG emissions, and how sensitive the climate is to greenhouse gases.[32] Scientists are pretty sure that with double the amount of GHG in the atmosphere the world would warm by 2.5 °C to 4 °C; but how much more humans will emit is less certain.[33] The projected magnitude of warming by 2100 is closely related to the level of cumulative emissions over the 21st century (total emissions between 2000 and 2100). The higher the cumulative emissions over this time period, the greater the level of warming is projected to occur.[34]
If emissions of CO2 were to be abruptly stopped and no negative emission technologies deployed, the Earth's climate would not start moving back to its pre-industrial state. Instead, temperatures would stay elevated at the same level for several centuries. After about a thousand years, 20% to 30% of human-emitted CO2 will remain in the atmosphere, not taken up by the ocean or the land, committing the climate to a warmer state long after emissions have stopped.[35]
Mitigation policies currently in place will result in about 2.7 °C (2.0–3.6 °C, depending on how sensitive the climate is to greenhouse gas emissions) warming above pre-industrial levels. If all unconditional pledges and targets made by governments are achieved the temperature will rise by around 2.4 °C. If additionally all the countries that adopted or are considering to adopt net-zero targets will achieve it the temperature will rise by a median of 1.8 °C. There is a substantial gap between national plans and commitments and actions so far taken by governments around the world.[36]
Weather-related changes

For the most part, weather continues much as it has, except climate change makes many aspects more extreme, especially heat waves, drought, rain intensity, and storm size and intensity. That is, climate change does not create these events, it exacerbates them.[38][39] Global warming leads to an increase in extreme weather events such as heat waves, droughts, cyclones, blizzards and rainstorms.[40] Such events will continue to occur more often and with greater intensity.[41] Some individual extreme weather events are made worse by climate change.[42]
The lower and middle atmosphere, where nearly all of the weather occurs, are heating due to the enhanced greenhouse effect. Increased greenhouse gases cause the higher parts of the atmosphere, the stratosphere, to cool.[43] The heated atmosphere contains more water vapour, which is also a greenhouse gas and acts as a self-reinforcing feedback.[44]
As temperatures increase, so does evaporation and atmospheric moisture content that increases heating by trapping outgoing radiation. Excess water vapour gets caught up in storms and makes them more intense, larger, and potentially longer lasting, so that rain and snow events are stronger and increase risk of flooding. Extra drying worsens any natural dry spells and droughts, and increases risk of heat waves and wildfires.[45]
Rain and snow
Warming by greenhouse gas forcing has increased contrasts in rainfall amounts between wet and dry seasons, meaning "wet seasons are getting wetter, dry seasons are getting drier".[46]: 8 Over oceans, salty areas are getting saltier and other regions are becoming less saline.[47] Warming has also resulted in a detectable increase in the precipitation of northern high latitudes.[46]: 8
Higher temperatures lead to increased evaporation and surface drying. As the air warms, its water-holding capacity also increases, particularly over the oceans. Air can hold 7% more water vapour for every degree Celsius it is warmed.[46]: 8 Changes have already been observed in the amount, intensity, frequency, and type of precipitation. Widespread increases in heavy precipitation have occurred even in places where total rain amounts have decreased.[48]
In the case of precipitation, the rising temperatures and extra heat will "intensify" the Earth's water cycle, which means it will increase evaporation. Increased atmospheric water vapour results in more frequent and intense downpours and causes stronger extended droughts in certain regions. As a result, storm-affected areas are likely to experience increases in precipitation and an increased risk of flooding. In contrast, areas far away from storm tracks are likely to experience less precipitation and an increased risk of drought. Overall, there is likely to be longer hot dry spells, broken by more intense heavy rainfalls (along the lines of the English proverb "it never rains but it pours!").[9]: 151
Changes in regional climate are expected to include greater temperature increases over land, with most warming at high northern latitudes, and least warming over the Southern Ocean and parts of the North Atlantic Ocean.[49] Future changes in precipitation are expected to follow existing trends, with reduced precipitation over subtropical land areas, and increased precipitation at subpolar latitudes and some equatorial regions.[50]
Lightnings
Lightning strikes occur more frequently in hot weather. According to a study published in 2014, the number of lightning strikes will increase by about 12% for every degree of rise in global average air temperature.[51] However, the impact of global warming on lightning rates is poorly constrained.[51]
Heat waves and temperature extremes

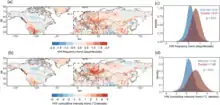
Global heating boosts the probability of extreme weather events such as heat waves[54][55] where the daily maximum temperature exceeds the average maximum temperature by 5 °C (9 °F) for more than five consecutive days.[56] In the last 30–40 years, heat waves with high humidity have become more frequent and severe. Extremely hot nights have doubled in frequency. The area in which extremely hot summers are observed has increased 50–100 fold. Heat waves with high humidity pose a big risk to human health while heat waves with low humidity lead to dry conditions that increase wildfires. The mortality from extreme heat is larger than the mortality from hurricanes, lightning, tornadoes, floods, and earthquakes together.[57]
It was estimated in 2013 that global warming had increased the probability of local record-breaking monthly temperatures worldwide by a factor of 5.[58] This was compared to a baseline climate in which no global warming had occurred. Using a medium global warming scenario, they project that by 2040, the number of monthly heat records globally could be more than 12 times greater than that of a scenario with no long-term warming.
Study results indicate that limiting global warming to 1.5 °C would prevent most of the tropics from reaching the wet-bulb temperature of the human physiological limit of 35 °C.[59][60]
Future climate change will include more very hot days and fewer very cold days.[61] The frequency, length and intensity of heat waves will very likely increase over most land areas.[61] Higher growth in anthropogenic GHG emissions would cause more frequent and severe temperature extremes.[62] Globally, cold waves have decreased in frequency.[52]: 8 There is some evidence climate change leads to a weakening of the polar vortex, which would make the jet stream more wavy.[63] This would lead to outbursts of very cold winter weather across parts of Eurasia[64] and North America, as well as very warm air incursions into the Arctic.[65][66][67]
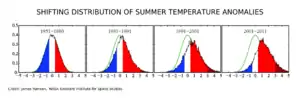
Tropical cyclones and storms
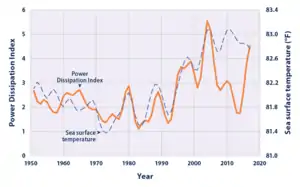
Extreme event attribution
Weather-related impacts
Floods

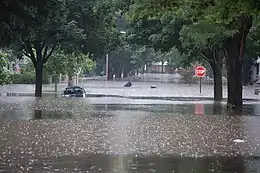
A warming climate will intensify rainfall events. When floods occur in this warmer future, these floods will be more severe.[46]: 1156 Some regions will experience an increased in flooding, some a decrease. This depends on several factors, such as changes in snowmelt, soil moisture and rainfall.[46]: 1156 Flooding can be in the form of urban flooding, river flooding or coastal flooding, Sea level rise further increases risks of coastal flooding: if sea levels rise by a further 0.15 m, 20% more people will be exposed to a 1 in a 100 year coastal flood, assuming no population growth and no further adaptation.[79] With an extra 0.75 m, this rises to a doubling of people exposed.[79]
Global warming makes bigger storm events more common due to an intensification of the water cycle.[80] This increase in the frequency of large storm events would alter existing Intensity-Duration-Frequency curves (IDF curves) due to the change in frequency, but also by lifting and steepening the curves in the future.[81]
Droughts
Climate change affects multiple factors associated with droughts, such as how much rain falls and how fast the rain evaporates again. Warming over land drives an increase in atmospheric evaporative demand which will increase the severity and frequency of droughts around much of the world.[46]: 1057 Scientists predict that in some regions of the world, there will be less rain in future due to global warming. These regions will therefore be more prone to drought in future: subtropical regions like the Mediterranean, southern Africa, south-western Australia and south-western South America, as well as tropical Central America, western Africa and the Amazon basin.[46]: 1157
Physics dictates that higher temperatures lead to increased evaporation. The effect of this is soil drying and increased plant stress which will have impacts on agriculture.[46]: 1157 For this reason, even those regions where large changes in precipitation are not expected (such as central and northern Europe) will experience soil drying.[46]: 1157 The latest prediction of scientists in 2022 is that "If emissions of greenhouse gases are not curtailed, about a third of global land areas are projected to suffer from at least moderate drought by 2100".[46]: 1157 When droughts occur they are likely to be more intense than in the past.[82]
Due to limitations on how much data is available about drought in the past, it is often impossible to confidently attribute a specific drought to human-induced climate change. Some areas however, such as the Mediterranean and California, already show the impacts of human activities.[83] Their impacts are made worse because of increased water demand, population growth, urban expansion, and environmental protection efforts in many areas.[84]
The frequency and the duration of droughts have both increased: They increased by 29% from the year 2000 to 2022.[85] The prediction is that by 2050 more than 75% of humanity will live in drought conditions.[85]: 37 Land restoration, especially by agroforestry, can help reduce the impact of droughts.[85]
In 2019 the Intergovernmental Panel on Climate Change issued a Special Report on Climate Change and Land. The main statements of the report include:[86][87] Between 1960 and 2013 the area of drylands in drought increased by 1% per year. In 2015, around 500 million people lived in areas impacted by desertification between the 1980s and 2000s. People who live in areas affected by land degradation and desertification are "increasingly negatively affected by climate change".
In the past, there were some apparently conflicting results in the scientific literature about how drought is changing due to climate change.[82] This was found to be mostly due to problems with collection and analysis of data, for example in the formulation of the Palmer Drought Severity Index (PDSI) and the data sets used to determine the evapotranspiration component. The accurate attribution of the causes of drought also requires accounting for natural variability, especially El Niño Southern Oscillation (ENSO) effects and better analysis of precipitation data.[82]
Wildfires

Globally, climate change promotes the type of weather that makes wildfires more likely. In some areas, an increase of wildfires has been attributed directly to climate change. That warmer climate conditions pose more risks of wildfire is consistent with evidence from Earth's past: there was more fire in warmer periods, and less in colder climatic periods.[89] Climate change increases evaporation, which can cause vegetation to dry out. When a fire starts in an area with very dry vegetation, it can spread rapidly. Higher temperatures can also make the fire season longer, the time period in which severe wildfires are most likely. In regions where snow is disappearing, the fire season may get particularly more extended.[90]
Even though weather conditions are raising the risks of wildfires, the total area burnt by wildfires has decreased globally. This is mostly the result of the conversion of savanna into croplands, after which there is less forest area that can burn. Prescribed burning, an indigenous practice in the US and Australia, can reduce the area burnt too, and may form an adaptation to increased risk.[90] The carbon released from wildfires can further increase greenhouse gas concentrations. This feedback is not yet fully integrated into climate models.[52]: 20
Oceans
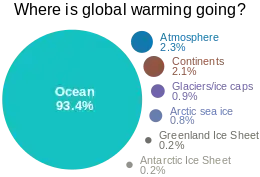
_and_annual_average_(black_line)_of_global_upper_ocean_heat_content_for_the_0-700m_layer_between_1955-2008.gif)
There are many significant effects of climate change on oceans including: an increase in sea surface temperature as well as ocean temperatures at greater depths, more frequent marine heatwaves, a reduction in pH value, a rise in sea level from ocean warming and ice sheet melting, sea ice decline in the Arctic, increased upper ocean stratification, reductions in oxygen levels, increased contrasts in salinity (salty areas becoming saltier and fresher areas becoming less salty),[93] changes to ocean currents including a weakening of the Atlantic meridional overturning circulation, and stronger tropical cyclones and monsoons.[94] All these changes have knock-on effects which disturb marine ecosystems. The root cause of these observed changes is the Earth warming due to anthropogenic emissions of greenhouse gases, such as for example carbon dioxide and methane. This leads inevitably to ocean warming, because the ocean is taking up most of the additional heat in the climate system.[95] Some of the additional carbon dioxide in the atmosphere is taken up by the ocean (via carbon sequestration), which leads to ocean acidification of the ocean water.[96] It is estimated that the ocean takes up roughly a quarter of total anthropogenic CO2 emissions.[96]
Warming of the ocean surface due to higher air temperatures leads to increased ocean temperature stratification.[97]: 471 The decline in mixing of the ocean layers stabilises warm water near the surface while reducing cold, deep water circulation. The reduced up and down mixing reduces the ability of the ocean to absorb heat, directing a larger fraction of future warming toward the atmosphere and land. Energy available for tropical cyclones [98]and other storms is expected to increase, nutrients for fish in the upper ocean layers are set to decrease, as is the capacity of the oceans to store carbon.[99]
Warmer water cannot contain as much oxygen as cold water. As a result, the gas exchange equilibrium changes to reduce ocean oxygen levels and increase oxygen in the atmosphere. Increased thermal stratification may lead to reduced supply of oxygen from the surface waters to deeper waters, and therefore further decrease the water's oxygen content.[100] The ocean has already lost oxygen throughout the water column, and oxygen minimum zones are expanding worldwide.[97]: 471
These changes disturb marine ecosystems, which can cause both extinctions and population explosions, change the distribution of species,[94] and impact coastal fishing and tourism. Increase of water temperature will also have a devastating effect on various oceanic ecosystems, such as coral reefs. The direct effect is the coral bleaching of these reefs, which live within a narrow temperature margin, so a small increase in temperature would have a drastic effect in these environments. Ocean acidification and temperature rise will also affect the productivity and distribution of species within the ocean, threatening fisheries and disrupting marine ecosystems. Loss of sea ice habitats due to warming will severely impact the many polar species which depend on this sea ice. Many of these climate change pressures interact, compounding the pressures on the climate system and on ocean ecosystems.[94].jpg.webp)

Ice and snow
The cryosphere, the area of the Earth covered by snow or ice, is extremely sensitive to changes in global climate.[103] Northern Hemisphere average annual snow cover has declined in recent decades. This pattern is consistent with warmer global temperatures. Some of the largest declines have been observed in the spring and summer months.[92] During the 21st century, snow cover is projected to continue its retreat in almost all regions.[104]
Glaciers and ice sheets decline
Since the beginning of the twentieth century, there has been a widespread retreat of glaciers.[105] The melting of the Greenland and West Antarctic ice sheets will continue to contribute to sea level rise over long time-scales.[106] The Greenland ice sheet loss is mainly driven by melt from the top, whereas Antarctic ice loss is driven by warm ocean water melting the outlet glaciers.[107] Research on Antarctica published in 2022, including the first map of iceberg calving, doubles the previous estimates of loss from ice shelves.[108][109]
Future melt of the West Antarctic ice sheet is potentially abrupt under a high emission scenario, as a consequence of a partial collapse.[110] Part of the ice sheet is grounded on bedrock below sea level, making it possibly vulnerable to the self-enhancing process of marine ice sheet instability. A further hypothesis is that marine ice cliff instability would also contribute to a partial collapse, but limited evidence is available for its importance.[111] A partial collapse of the ice sheet would lead to rapid sea level rise and a local decrease in ocean salinity. It would be irreversible on a timescale between decades and millennia.[110]
In contrast to the West Antarctic ice sheet, melt of the Greenland ice sheet is projected to be taking place more gradually over millennia.[110] Sustained warming between 1 °C (low confidence) and 4 °C (medium confidence) would lead to a complete loss of the ice sheet, contributing 7 m to sea levels globally.[112] The ice loss could become irreversible due to a further self-enhancing feedback: the elevation-surface mass balance feedback. When ice melts on top of the ice sheet, the elevation drops. As air temperature is higher at lower altitude, this promotes further melt.[113]
Sea ice changes
Sea ice reflects 50% to 70% of the incoming solar radiation, while 6% of the incoming solar energy is reflected by the ocean. With less solar energy, the sea ice absorbs and holds the surface colder, which can be a positive feedback toward climate change.[114] As the climate warms, snow cover and sea ice extent decrease. Large-scale measurements of sea-ice have only been possible since the satellite era. The age of the sea ice is an important feature of the state of the sea ice cover.[115]
Arctic sea ice decline
Arctic sea ice began to decline at the beginning of the twentieth century but the rate is accelerating. Since 1979, satellite records indicate the decline in summer sea ice coverage has been about 13% per decade.[116][117] The thickness of sea ice has also decreased by 66% or 2.0 m over the last six decades with a shift from permanent ice to largely seasonal ice cover.[118] While ice-free summers are expected to be rare at 1.5 °C degrees of warming, they are set to occur at least once every decade at a warming level of 2.0 °C.[119] The Arctic will likely become ice-free at the end of some summers before 2050.[107]: 9
Sea ice changes in Antarctica
The annual mean Antarctic sea ice area is not showing a clear downwards or upwards trend over the period of reliable satellite data starting in 1979.[107]: 1251 There are remaining uncertainties on how to interpret this data due to the limited observational records and discrepancies between modelled and observed results for the sea ice cover..[107]: 1253
Permafrost thawing
The southern part of the Arctic region (home to 4 million people) has experienced a temperature rise of 1 °C to 3 °C (1.8 °F to 5.4 °F) over the last 50 years.[120] Canada, Alaska and Russia are experiencing initial melting of permafrost. This may disrupt ecosystems and by increasing bacterial activity in the soil lead to these areas becoming carbon sources instead of carbon sinks.[121] Eastern Siberia's permafrost is gradually disappearing in the southern regions, leading to the loss of nearly 11% of Siberia's nearly 11,000 lakes since 1971.[122] At the same time, western Siberia is at the initial stage where melting permafrost is creating new lakes, which will eventually start disappearing as in the east. Furthermore, permafrost melting will eventually cause methane release from melting permafrost peat bogs.
Wildlife and nature
Recent warming has strongly affected natural biological systems.[11] Species worldwide are moving poleward to colder areas. On land, species may move to higher elevations, whereas marine species find colder water at greater depths.[12] Of the drivers with the biggest global impact on nature, climate change ranks third over the five decades before 2020, with only change in land use and sea use, and direct exploitation of organisms having a greater impact.[129]
The impacts of climate change in nature and nature's contributions to humans are projected to become more pronounced in the next few decades.[130] Examples of climatic disruptions include fire, drought, pest infestation, invasion of species, storms, and coral bleaching events. The stresses caused by climate change, added to other stresses on ecological systems (e.g. land conversion, land degradation, harvesting, and pollution), threaten substantial damage to or complete loss of some unique ecosystems, and extinction of some critically endangered species.[131][132][133] Key interactions between species within ecosystems are often disrupted because species from one location do not move to colder habitats at the same rate, giving rise to rapid changes in the functioning of the ecosystem.[12] Impacts include changes in regional rainfall patterns, earlier leafing of trees and plants over many regions; movements of species to higher latitudes and altitudes in the Northern Hemisphere; changes in bird migrations in Europe, North America and Australia; and shifting of the oceans' plankton and fish from cold- to warm-adapted communities.[134]
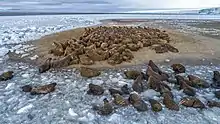
Ecosystems on land
Climate change has been estimated to be a major driver of biodiversity loss in cool conifer forests, savannas, mediterranean-climate systems, tropical forests, and the Arctic tundra.[136] In other ecosystems, land-use change may be a stronger driver of biodiversity loss, at least in the near-term.[136] Beyond the year 2050, climate change may be the major driver for biodiversity loss globally.[136] Climate change interacts with other pressures such as habitat modification, pollution and invasive species. Interacting with these pressures, climate change increases extinction risk for a large fraction of terrestrial and freshwater species.[137] Between 1% and 50% of species in different groups were assessed to be at substantially higher risk of extinction due to climate change.[13]
Amazon rainforest

Rainfall that falls on the Amazon rainforest is recycled when it evaporates back into the atmosphere instead of running off away from the rainforest. This water is essential for sustaining the rainforest. Due to deforestation the rainforest is losing this ability, exacerbated by climate change which brings more frequent droughts to the area. The higher frequency of droughts seen in the first two decades of the 21st century, as well as other data, signal that a tipping point from rainforest to savanna might be close. One study concluded that this ecosystem could enter a mode of a 50-years-long collapse to a savanna around 2021, after which it would become increasingly and disproportionally more difficult to prevent or reverse this shift.[139][140][141]
Marine ecosystems
Marine heatwaves have seen an increased frequency and have widespread impacts on life in the oceans, such as mass dying events and coral bleaching.[142] Harmful algae blooms have increased in response to warming waters, loss of oxygen and eutrophication.[143] Between one-quarter and one-third of our fossil fuel emissions are consumed by the earth's oceans, which are now 30 percent more acidic than they were in pre-industrial times. This acidification poses a serious threat to aquatic life, particularly creatures such as oysters, clams, and coral with calcified shells or skeletons.[135] Melting sea ice destroys habitat, including for algae that grows on its underside.[144] It is likely that the oceans warmed faster between 1993 and 2017 compared to the period starting in 1969.[145]
Warm water coral reefs are very sensitive to global warming and ocean acidification. Coral reefs provide a habitat for thousands of species and ecosystem services such as coastal protection and food. The resilience of reefs can be improved by curbing local pollution and overfishing, but 70–90% of today's warm water coral reefs will disappear even if warming is kept to 1.5 °C.[14] Coral reefs are not the only framework organisms, organisms that build physical structures that form habitats for other sea creatures, affected by climate change: mangroves and seagrass are considered to be at moderate risk for lower levels of global warming according to the Special Report on the Ocean and Cryosphere in a Changing Climate.[146]
Tipping points and irreversible impacts
Self-reinforcing feedbacks amplify climate change.[147] The climate system exhibits "threshold behaviour" or tipping points when these feedbacks lead parts of the Earth system into a new state, such as the runaway loss of ice sheets or the destruction of forests.[148][149] Tipping points are studied using data from Earth's distant past and by physical modelling.[148] There is already moderate risk of global tipping points at 1 °C above pre-industrial temperatures, and that risk becomes high at 2.5 °C.[150]
Tipping points are "perhaps the most 'dangerous' aspect of future climate changes", leading to irreversible impacts on society.[151] Many tipping points are interlinked, so that triggering one may lead to a cascade of effects,[152] even well below 2 °C of warming.[153] A 2018 study states that 45% of environmental problems, including those caused by climate change are interconnected and make the risk of a domino effect bigger.[154][155]
There are a number of climate change impacts on the environment that may be irreversible, at least over the timescale of many human generations.[156] These include the large-scale singularities such as the melting of the Greenland and West Antarctic ice sheets, and changes to the Atlantic Meridional Overturning Circulation.[156] In biological systems, the extinction of species would be an irreversible impact.[156] In social systems, unique cultures may be lost[156] or the survival of endangered languages may be exacerbated due to climate change.[157] For example, humans living on atoll islands face risks due to sea level rise, sea surface warming, and increased frequency and intensity of extreme weather events.[158][157]
Health, food security and water security
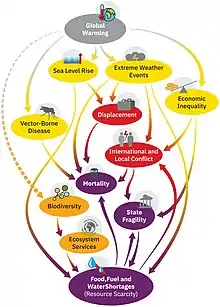
Health
The effects of climate change on human health include direct effects of extreme weather, leading to injury and loss of life,[160] as well as indirect effects, such as undernutrition brought on by crop failures or a lack of access to safe drinking water.[161] Climate change poses a wide range of risks to population health.[162] The three main categories of health risks include: (i) direct-acting effects (e.g. due to heat waves, extreme weather disasters), (ii) impacts mediated via climate-related changes in ecological systems and relationships (e.g. crop yields, mosquito ecology, marine productivity), and (iii) the more diffuse (indirect) consequences relating to impoverishment, displacement, and mental health problems.
More specifically, the relationship between health and heat (increased global temperatures) includes the following aspects:[163] exposure of vulnerable populations to heatwaves, heat-related mortality, impacts on physical activity and labour capacity and mental health. There is a range of climate-sensitive infectious diseases which may increase in some regions, such as mosquito-borne diseases, diseases from vibrio pathogens, cholera and some waterborne diseases.[163] Health is also acutely impacted by extreme weather events (floods, hurricanes, droughts, wildfires) through injuries, diseases and air pollution in the case of wildfires. Other health impacts from climate change include migration and displacement due rising sea levels; food insecurity and undernutrition,[163] reduced availability of drinking water, increased harmful algal blooms in oceans and lakes and increased ozone levels as an additional air pollutant during heatwaves.[164]Food security
Climate change will impact agriculture and food production around the world due to the effects of elevated CO2 in the atmosphere; higher temperatures; altered precipitation and transpiration regimes; increased frequency of extreme events; and modified weed, pest, and pathogen pressure.[165] Droughts result in crop failures and the loss of pasture for livestock.[166] Loss and poor growth of livestock cause milk yield and meat production to decrease.[167] The rate of soil erosion is 10–20 times higher than the rate of soil accumulation in agricultural areas that use no-till farming. In areas with tilling it is 100 times higher. Climate change makes this type of land degradation and desertification worse.[168]
Climate change is projected to negatively affect all four pillars of food security: not only how much food is available, but also how easy food is to access (prices), food quality and how stable the food system is.[169] For example, climate change is already affecting the productivity of wheat and other key staples.[170][171] The availability, quality and stability of wines are impacted by shifts in temperature affecting the traditional range and practices of viniculture, and by smoke taint from extreme fire events.[172]
In many areas, fisheries have already seen their catch decrease because of global warming and changes in biochemical cycles. In combination with overfishing, warming waters decrease the maximum catch potential.[3]: 12 Global catch potential is projected to reduce further in 2050 by less than 4% if emissions are reduced strongly, and by about 8% for very high future emissions, with growth in the Arctic Ocean.[173]
Water security
Between 1.5 and 2.5 billion people live in areas with regular water security issues. If global warming would reach 4 °C, water insecurity would affect about twice as many people.[174] Water resources are projected to decrease in most dry subtropical regions and mid-latitudes, but increase in high latitudes. However, as streamflow becomes more variable, even regions with increased water resources can experience additional short-term shortages.[175] The arid regions of India, China, the US and Africa are already seeing dry spells and drought impact water availability.[174]
Water resources can be affected by climate change in various ways. The total amount of freshwater available can change, for instance due to dry spells or droughts. Heavy rainfall and flooding can have an impact on water quality: pollutants can be transported into water bodies by the increased surface runoff. In coastal regions, more salt may find its way into water resources due to higher sea levels and more intense storms. Higher temperatures also directly degrade water quality: warm water contains less oxygen.[174]
Water-related impacts from climate change impact people's water security on a day-to-day basis. They include: increased frequency and intensity of heavy precipitation, accelerated melting of glaciers, changes in frequency, magnitude, and timing of floods; more frequent and severe droughts in some places; decline in groundwater storage, and reduction in groundwater recharge and water quality deterioration due to extreme events.[176]: 4–8 Water resources can be affected by climate change in various ways. The total amount of locally available freshwater available can change, for instance due to dry spells or droughts. There can also be reduced water quality due to the effects of climate change.
Global climate change is "likely to increase the complexity and costs of ensuring water security".[177] It creates new threats and adaptation challenges.[178] This is because climate change leads to increased hydrological variability and extremes. Climate change has many impacts on the water cycle, resulting in higher climatic and hydrological variability, which means that water security will be compromised.[179]: vII Changes in the water cycle threaten existing water infrastructure and make it harder to plan future investments that can cope with uncertain changes in hydrologic variability.[178] This makes societies more vulnerable to extreme water-related events and therefore increases water insecurity.[179]: vIIEconomic impacts
Economic forecasts of the impact of global warming vary considerably. Researchers have warned that current economic modelling may seriously underestimate the impact of potentially catastrophic climate change, and point to the need for new models that give a more accurate picture of potential damages. Nevertheless, one 2018 study found that potential global economic gains if countries implement mitigation strategies to comply with the 2 °C target set at the Paris Agreement are in the vicinity of US$17 trillion per year up to 2100 compared to a very high emission scenario.[180]
Global losses reveal rapidly rising costs due to extreme weather events since the 1970s.[40] Socio-economic factors have contributed to the observed trend of global losses, such as population growth and increased wealth.[181] Part of the growth is also related to regional climatic factors, e.g., changes in precipitation and flooding events. It is difficult to quantify the relative impact of socio-economic factors and climate change on the observed trend.[182] The trend does, however, suggest increasing vulnerability of social systems to climate change.[182]
Economic inequality
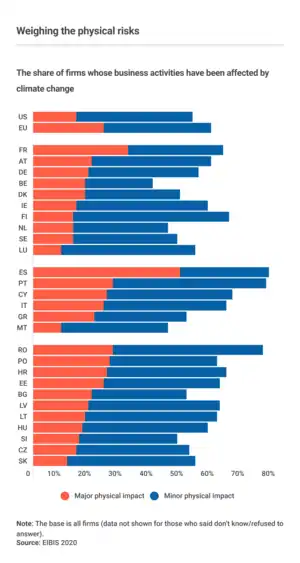
Climate change has contributed towards global economic inequality. Wealthy countries in colder regions have either felt little overall economic impact from climate change, or possibly benefited, whereas poor hotter countries very likely grew less than if global warming had not occurred.[183][184]
The total economic impacts from climate change are difficult to estimate, but increase for higher temperature changes.[185] For instance, total damages are estimated to be 90% less if global warming is limited to 1.5 °C compared to 3.66 °C, a warming level chosen to represent no mitigation.[186] One study found a 3.5% reduction in global GDP by the end of the century if warming is limited to 3 °C, excluding the potential effect of tipping points. Another study noted that global economic impact is underestimated by a factor of two to eight when tipping points are excluded from consideration.[186] In the Oxford Economics high emission scenario, a temperature rise of 2 degrees by 2050 would reduce global GDP by 2.5% – 7.5%. By the year 2100 in this case, the temperature would rise by 4 degrees, which could reduce the global GDP by 30% in the worst case.[187]
Most affected sectors apart from agriculture and fisheries
Thermal power stations (fossil fuel plants and nuclear power plants) depend on water to cool them. Not only is there increased demand for fresh water, but climate change can increase the likelihood of drought and fresh water shortages. Another impact for thermal power plants, is that increasing the temperatures in which they operate reduces their efficiency and hence their output.[188] Changes in the amount of river flow correlate with the amount of energy produced by a dam. The result of diminished river flow can be a power shortage in areas that depend heavily on hydroelectric power. Brazil in particular, is vulnerable due to its having reliance on hydroelectricity as increasing temperatures, lower water flow, and alterations in the rainfall regime, could reduce total energy production by 7% annually by the end of the century.[188]
Oil and natural gas infrastructure is affected by the effects of climate change and the increased risk of disasters such as storm, cyclones, flooding and rising sea levels.[189]
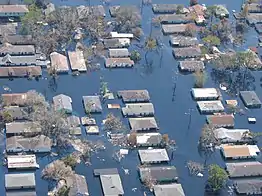
Insurance is an important tool to manage risks, but often unavailable to poorer households. Due to climate change, premiums are going up for certain types of insurance, such as flood insurance. Poor adaptation to climate change further widens the gap between what people can afford and the costs of insurance, as risks increase.[190] In 2019, Munich Re noted that climate change could cause home insurance to become unaffordable for households at or below average incomes.[191]
Roads, airport runways, railway lines and pipelines, (including oil pipelines, sewers, water mains etc.) may require increased maintenance and renewal as they become subject to greater temperature variation. Regions already adversely affected include areas of permafrost, which are subject to high levels of subsidence, resulting in buckling roads, sunken foundations, and severely cracked runways.[192]
Impacts on societies
Climate change impacts health, the availability of drinking water and food, inequality and economic growth. The effects of climate change are often interlinked and can exacerbate each other as well as existing vulnerabilities.[193][194][195] The impacts are often exacerbated by related environmental disruptions and pressures such as pollution and biodiversity loss.[196] Some areas may become too hot for humans to live in.[197][198] People in some areas may experience internal or long-distance displacement (and thus become climate refugees) triggered by climate change related changes or disasters.
The effects of climate change, in combination with the sustained increases in greenhouse gas emissions, have led scientists to characterize it as a "climate emergency" or "climate crisis".[199][200][201] Some climate researchers[202][203] and activists[204] have called it an "existential threat to civilization".
Displacement and migration
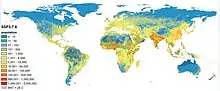
Climate change affects displacement of people in several ways. Firstly, involuntary displacement may increase through the increased number and severity of weather-related disasters which destroy homes and habitats. Effects of climate change such as desertification and rising sea levels gradually erode livelihood and force communities to abandon traditional homelands for more accommodating environments. On the other hand, some households may fall (further) into poverty due to climate change, limiting their ability to move to areas less affected.[205]
According to the Internal Displacement Monitoring Centre in 2020 approximately 30 million people were displaced by extreme weather events while approximately 10 million by violence and wars and climate change significantly contributed to this.[206][207] The United Nations says there are already 64 million migrants in the world fleeing wars, hunger, persecution and the effects of global warming.[208] In 2018, the World Bank estimated that climate change will cause internal migration of between 31 and 143 million people as they escape crop failures, water scarcity, and sea level rise. The study only included Sub-Saharan Africa, South Asia, and Latin America.[209][210]
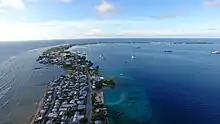
Asia and the Pacific is the global area most prone to natural disasters, both in terms of the absolute number of disasters and of populations affected. It is highly exposed to climate impacts, and is home to highly vulnerable population groups, who are disproportionately poor and marginalized. A 2015 Asian Development Bank report highlights "environmental hot spots" that are particular risk of flooding, cyclones, typhoons, and water stress.[211]
Gradual but pervasive environmental change and sudden natural disasters both influence the nature and extent of human migration but in different ways. United Nations High Commissioner for Refugees stated that climate change increases mass displacement, in many regions, including Sahel, East Africa, South Asia, the "drought corridor" in Latin America. 90% of refugees comes from "climate vulnerable hotspots".[212]
Some Pacific Ocean island nations, such as Tuvalu, Kiribati, and the Maldives,[213] are considering the eventual possibility of evacuation, as flood defense may become economically unrealistic.
The two broad sets of potential interventions are climate change mitigation and building "greater capacity within vulnerable populations so that they may adapt through means that do not lead to distress migration or conflict".[214] There are various reasons for why, especially large-scale- or distant-source-, migration is often widely undesired and opposed in both (or either) host and origin countries, such as shortage of housing,[215] cultural identity or cohesion[216] or sovereignty, and economics-related reasons.
Governments have considered various approaches to reduce migration compelled by environmental conditions in at-risk communities, including programs of social protection, livelihoods development, basic urban infrastructure development, and disaster risk management. Some experts support migration as an appropriate way for people to cope with environmental changes. However, this is controversial because migrants – particularly low-skilled ones – are among the most vulnerable people in society and are often denied basic protections and access to services.[211]
Slow-onset disasters and gradual environmental erosion such as desertification, reduction of soil fertility, coastal erosion and sea-level rise are likely to induce long-term migration.[217] Migration related to desertification and reduced soil fertility is likely to be predominantly from rural areas in developing countries to towns and cities.[218]
Conflict
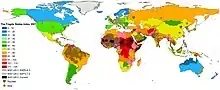
Climate change can worsen conflicts by exacerbating tensions over limited resources like drinking water (in the case of water conflicts). Climate change also has the potential to cause large population dislocations and migration, which can also lead to increased tensions.[219][220] However, factors other than climate change are judged to be substantially more important in affecting conflict. These factors include intergroup inequality and low socio-economic development.[221] In some cases, climate change can even lead to more peaceful relationships between groups, as environmental problems requires common policy to be developed.[222]
Global warming has been described as a "threat multiplier".[223] Certain conditions make it more likely that climate change impacts conflict: ethnic exclusion, an economy dependent on agriculture, insufficient infrastructure, poor local governance, and low levels of development.[222] A spike in wheat prices following crop losses from a period of drought may have contributed to the onset of the "Arab Spring" protests and revolutions in 2010.[224][222]
Social impacts on vulnerable groups
The impacts of climate change on humans are not distributed uniformly within communities. Individual and social factors such as gender, age, education, ethnicity, geography and language lead to differential vulnerability and capacity to adapt to the effects of climate change. The following more vulnerable groups have been identified:
- People living in poverty: Climate change disproportionally affects poor people in low-income communities and developing countries around the world. Those in poverty have a higher chance of experiencing the ill-effects of climate change due to the increased exposure and vulnerability.[225] A 2020 World Bank paper estimated that between 32 million to 132 million additional people will be pushed into extreme poverty by 2030 due to climate change.[226]
- Women: Climate change increases gender inequality,[227] reduces women's ability to be financially independent,[228] and has an overall negative impact on the social and political rights of women, especially in economies that are heavily based on agriculture.[227]
- Indigenous peoples: Indigenous communities geographically tend to be located in regions more vulnerable to climate change such as native rainforests, the Arctic, and coastal areas.[229] Indigenous communities across the globe generally have economic disadvantages that are not as prevalent in non-indigenous communities due to the ongoing oppression they have experienced. These disadvantages include lower education levels and higher rates of poverty and unemployment, which add to their vulnerability to climate change.[230]
- Children: The Lancet review on health and climate change lists children as the worst-affected category by climate change.[231] Children are also 14–44 percent more likely to die from environmental factors,[232] again leaving them the most vulnerable. Those in urban areas will be affected by lower air quality and overcrowding, and will struggle the most to better their situation.[233]
- Racial minorities: The environmental justice (EJ) movement and climate justice (CJ) movement address environmental racism in bringing attention and enacting change so that marginalized populations are not disproportionately vulnerable to climate change and pollution.[234][235]
Human settlements
A major challenge for human settlements is sea level rise, indicated by ongoing observation and research of rapid declines in ice-mass balance from both Greenland and Antarctica. Estimates for 2100 are at least twice as large as previously estimated by IPCC AR4, with an upper limit of about two meters.[236] Depending on regional changes, increased precipitation patterns can cause more flooding or extended drought stresses water resources.
A 2020 study projects that regions inhabited by a third of the human population could become as hot as the hottest parts of the Sahara within 50 years without a change in patterns of population growth and without migration, unless greenhouse gas emissions are reduced. The projected annual average temperature of above 29 °C for these regions would be outside the "human temperature niche" – a suggested range for climate biologically suitable for humans based on historical data of mean annual temperatures (MAT) – and the most affected regions have little adaptive capacity as of 2020.[237][238]
In small islands and megadeltas, inundation as a result of sea level rise is expected to threaten vital infrastructure and human settlements.[239][240] This could lead to issues of statelessness for populations in countries such as the Maldives and Tuvalu[241] and homelessness in countries with low-lying areas such as Bangladesh.
Projections for cities in 2050
In 2019 the Crowther Lab from ETH Zürich paired the climatic conditions of 520 major cities worldwide with the predicted climatic conditions of cities in 2050. 22% of the major cities are predicted to have climatic conditions that do not exist in any city today. 2050 London will have a climate similar to 2019 Melbourne, Athens and Madrid like Fez, Morocco, Nairobi like Maputo. The Indian city Pune will be like Bamako in Mali, Bamako will be like Niamey in Niger. Brasilia will be like Goiania.[242][243]
Increased extreme heat exposure from both climate change and the urban heat island effect threatens urban settlements.[244]
Especially affected regions
The Arctic, Africa, small islands, Asian megadeltas and the Middle East are regions that are likely to be especially affected by climate change.[245][246] Low-latitude, less-developed regions are at most risk of experiencing negative impacts due to climate change.[17]
The ten countries of the Association of Southeast Asian Nations (ASEAN) are among the most vulnerable in the world to the negative effects of climate change, however, ASEAN's climate mitigation efforts are not commensurate with the climate change threats the region faces.[247] Africa is one of the most vulnerable continents to climate variability and change because of multiple existing stresses and low adaptive capacity. Climate change is projected to decrease freshwater availability in central, south, east and southeast Asia, particularly in large river basins. With population growth and increasing demand from higher standards of living, this decrease could adversely affect more than a billion people by the 2050s. Small islands, whether located in the tropics or higher latitudes, are already exposed to extreme weather events and changes in sea level. This existing exposure will likely make these areas sensitive to the effects of climate change.
Developed countries are also vulnerable to climate change, and have already been negatively affected by increases in the severity and frequency of some extreme weather events, such as heat waves, floods, wildfires, and tropical cyclones.[248][249][250]
Low-lying coastal regions
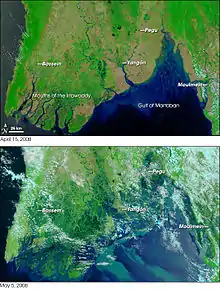
For historical reasons to do with trade, many of the world's largest and most prosperous cities are on the coast. In developing countries, the poorest often live on floodplains, because it is the only available space, or fertile agricultural land. These settlements often lack infrastructure such as dykes and early warning systems. Poorer communities also tend to lack the insurance, savings, or access to credit needed to recover from disasters.
Socioeconomic impacts of climate change in coastal and low-lying areas will be overwhelmingly adverse. The following impacts were projected in 2007 with very high confidence:[251]
- Coastal and low-lying areas would be exposed to increasing risks including coastal erosion due to climate change and sea level rise.
- By the 2080s, millions of people would experience floods every year due to sea level rise. The numbers affected were projected to be largest in the densely populated and low-lying mega-deltas of Asia and Africa; and smaller islands were judged to be especially vulnerable.
Given high coastal population density, estimates of the number of people at risk of coastal flooding from climate-driven sea-level rise varies from 190 million,[252] to 300 million or even 640 million in a worst-case scenario related to the instability of the Antarctic ice sheet.[253][254] The most people affected are in the densely-populated and low-lying megadeltas of Asia and Africa.[255]
The Greenland ice sheet is estimated to have reached a point of no return, continuing to melt even if warming stopped. Over time that would submerge many of the world's coastal cities including low-lying islands, especially combined with storm surges and high tides.[256]
Small islands
Small islands developing states are especially vulnerable to the effects of climate change, especially sea level rise. They are expected to experience more intense storm surges, salt water intrusion, and coastal destruction.[257] Low-lying small islands in the Pacific, Indian, and Caribbean regions are at risk of permanent inundation and population displacement.[258][259][260] On the islands of Fiji, Tonga and western Samoa, concentrations of migrants from outer islands inhabit low and unsafe areas along the coasts.[260]
Atoll nations, which include countries that are composed entirely of the smallest form of islands, called motus, are at risk of entire population displacement.[261][258] These nations include Kiribati, Maldives, the Marshall Islands, Tokelau, and Tuvalu.[258][259] Vulnerability is increased by small size, isolation from other land, low financial resources, and lack of protective infrastructure.[258]
A study that engaged the experiences of residents in atoll communities found that the cultural identities of these populations are strongly tied to these lands.[262] Human rights activists argue that the potential loss of entire atoll countries, and consequently the loss of national sovereignty, self-determination, cultures, and indigenous lifestyles cannot be compensated for financially.[261][258] Some researchers suggest that the focus of international dialogues on these issues should shift from ways to relocate entire communities to strategies that instead allow for these communities to remain on their lands.[261][262]
Possibility of societal collapse
Several researchers have suggested that collapse of the current societal organization – the contemporary global, interconnected civilization – could occur at 3 degrees of warming, especially when considering that climate change is not the only environmental pressure and challenge humanity faces.[263][264][265]
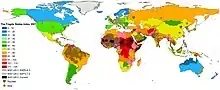
In 2022, researchers around the Centre for the Study of Existential Risk reported that the risk of climate change (indirectly) resulting in worldwide societal collapse, or possibly eventual human extinction, is a "dangerously underexplored" global topic, despite there being indications of such being possible as worst-case scenarios and that "integrated catastrophe assessment" is missing.[269][159]
Human impacts as rationale for mitigation
There is a misconception that climate change mitigation primarily is about conservation of nature (e.g. due to "love of nature"[270][271]) or the protection of the planet (which has experienced several mass extinction events) or an external environment – instead the primary rationale or intended effects and purpose of climate change mitigation is suggested to be protection of humans (from suffering, reduced quality of life and earlier death) and of sustained human civilization (e.g. national security) or, broadly, to enable a just future for all on a thriving planet which humans not only often value but also depend upon. It has been described as protection of humanity rather than protection of the climate.[272][273][274]
See also
- Anthropocene
- Developing countries (climate change section)
- Global catastrophic risk
- Politics of climate change
References
- "The Causes of Climate Change". climate.nasa.gov. NASA. Archived from the original on 21 December 2019.
- "Climate Science Special Report / Fourth National Climate Assessment (NCA4), Volume I". science2017.globalchange.gov. U.S. Global Change Research Program. Archived from the original on 14 December 2019.
- IPCC, 2019: Summary for Policymakers. In: IPCC Special Report on the Ocean and Cryosphere in a Changing Climate [H.-O. Pörtner, D.C. Roberts, V. Masson-Delmotte, P. Zhai, M. Tignor, E. Poloczanska, K. Mintenbeck, A. Alegría, M. Nicolai, A. Okem, J. Petzold, B. Rama, N.M. Weyer (eds.)]. Cambridge University Press, Cambridge, UK and New York, NY, USA. https://doi.org/10.1017/9781009157964.001.
- "The Study of Earth as an Integrated System". nasa.gov. NASA. 2016. Archived from the original on 2 November 2016.
- Oppenheimer, M., et al., Section 19.7.1: Relationship between Adaptation Efforts, Mitigation Efforts, and Residual Impacts, in: Chapter 19: Emergent risks and key vulnerabilities (archived 20 October 2014), pp.1080–1085, in IPCC AR5 WG2 A 2014
- Oppenheimer, M., et al., Section 19.6.2.2. The Role of Adaptation and Alternative Development Pathways, in: Chapter 19: Emergent risks and key vulnerabilities (archived 20 October 2014), pp.1072–1073, in IPCC AR5 WG2 A 2014
- "The Effects of Climate Change". NASA.gov. Archived from the original on 21 February 2022.
- Lindsey, Rebecca; Dahlman, Luann (28 June 2022). "Climate Change: Global Temperature". climate.gov. National Oceanographic and Atmospheric Administration. Archived from the original on 17 September 2022.
- Trenberth, Kevin E. (2022). The Changing Flow of Energy Through the Climate System (1 ed.). Cambridge University Press. doi:10.1017/9781108979030. ISBN 978-1-108-97903-0. S2CID 247134757.
- IPCC SRCCL Summary for Policymakers. 2019. p. 9.
- Rosenzweig; et al., "Chapter 1: Assessment of Observed Changes and Responses in Natural and Managed Systems", IPCC AR4 WG2 2007, Executive summary, archived from the original on 23 December 2018, retrieved 28 December 2018
- Pecl, Gretta T.; Araújo, Miguel B.; Bell, Johann D.; Blanchard, Julia; Bonebrake, Timothy C.; Chen, I.-Ching; Clark, Timothy D.; Colwell, Robert K.; Danielsen, Finn; Evengård, Birgitta; Falconi, Lorena (31 March 2017). "Biodiversity redistribution under climate change: Impacts on ecosystems and human well-being". Science. 355 (6332): eaai9214. doi:10.1126/science.aai9214. hdl:10019.1/120851. ISSN 0036-8075. PMID 28360268. S2CID 206653576. Archived from the original on 20 December 2019. Retrieved 11 January 2020.
- Settele, J.; Scholes, R.; Betts, R.; Bunn, S.; et al. (2014). "Chapter 4: Terrestrial and Inland Water Systems" (PDF). IPCC AR5 WG2 A 2014. p. 300. Archived (PDF) from the original on 19 December 2019. Retrieved 2 January 2020.
- Hoegh-Guldberg, O.; Jacob, D.; Taylor, M.; Bindi, M.; et al. (2018). "Chapter 3: Impacts of 1.5ºC Global Warming on Natural and Human Systems" (PDF). IPCC SR15 2018. p. 179. Archived (PDF) from the original on 15 November 2019. Retrieved 15 December 2019.
- Director, International (15 October 2018). "The Industries and Countries Most Vulnerable to Climate Change". International Director. Archived from the original on 2 January 2020. Retrieved 15 December 2019.
- Hoegh-Guldberg, O.; Jacob, D.; Taylor, M.; Bindi, M.; et al. (2018). "Chapter 3: Impacts of 1.5ºC Global Warming on Natural and Human Systems" (PDF). IPCC SR15 2018. pp. 212–213, 228, 252. Archived (PDF) from the original on 15 November 2019. Retrieved 15 December 2019.
- Schneider, S.H.; et al., "Ch 19: Assessing Key Vulnerabilities and the Risk from Climate Change", In IPCC AR4 WG2 2007, p. 796, Distribution of Impacts, in: Sec 19.3.7 Update on 'Reasons for Concern', archived from the original on 23 December 2018, retrieved 28 December 2018
- Wilbanks, T.J.; et al., "Ch 7: Industry, Settlement and Society", IPCC AR4 WG2 2007, pp. 373–376, Sec 7.4.2.5 Social issues and Sec 7.4.3 Key vulnerabilities, archived from the original on 23 December 2018, retrieved 28 December 2018
- IPCC SRCCL Summary for Policymakers. 2019. p. 7.
- Climate Change Is Already Driving Mass Migration Around the Globe Archived 18 December 2019 at the Wayback Machine, Natural Resources Defense Council, 25 January 2019
- Kennedy, John; Ramasamy, Selvaraju; Andrew, Robbie; Arico, Salvatore; Bishop, Erin; Braathen, Geir (2019). WMO statement on the State of the Global Climate in 2018. Geneva: Chairperson, Publications Board, World Meteorological Organization. p. 6. ISBN 978-92-63-11233-0. Archived from the original on 12 November 2019. Retrieved 24 November 2019.
- IPCC, 2013: Summary for Policymakers. Archived 26 July 2019 at the Wayback Machine In: Climate Change 2013: The Physical Science Basis. Contribution of Working Group I to the Fifth Assessment Report of the Intergovernmental Panel on Climate Change p.20
- Davy, Richard; Esau, Igor; Chernokulsky, Alexander; Outten, Stephen; Zilitinkevich, Sergej (2017). "Diurnal asymmetry to the observed global warming". International Journal of Climatology. 37 (1): 79–93. Bibcode:2017IJCli..37...79D. doi:10.1002/joc.4688. ISSN 1097-0088.
- Schneider; et al., "Chapter 19: Assessing key vulnerabilities and the risk from climate change", Contribution of Working Group II to the Fourth Assessment Report of the Intergovernmental Panel on Climate Change, 2007, Sec. 19.3.1 Introduction to Table 19.1, archived from the original on 23 December 2018, retrieved 28 December 2018, in IPCC AR4 WG2 2007.
- Joyce, Christopher (30 August 2018). "To Predict Effects Of Global Warming, Scientists Looked Back 20,000 Years". NPR. Archived from the original on 29 December 2019. Retrieved 29 December 2019.
- Overpeck, J.T. (20 August 2008), NOAA Paleoclimatology Global Warming – The Story: Proxy Data, NOAA Paleoclimatology Program – NCDC Paleoclimatology Branch, archived from the original on 3 February 2017, retrieved 20 November 2012
- The 20th century was the hottest in nearly 2,000 years, studies show Archived 25 July 2019 at the Wayback Machine, 25 July 2019
- Jansen, E.; Overpeck, J.; Briffa, K. R.; Duplessy, J.-C.; et al. "Chapter 6: Palaeoclimate". In IPCC AR4 WG1 2007. Sec. 6.3.2 What Does the Record of the Mid-Pliocene Show?. Archived from the original on 23 December 2018. Retrieved 28 December 2018.
- Oppenheimer, M.; Glavovic, B.; Hinkel, J.; van de Wal, R.; et al. (2019). "Chapter 4: Sea Level Rise and Implications for Low Lying Islands, Coasts and Communities" (PDF). IPCC SROCC 2019. p. 323. Archived (PDF) from the original on 20 December 2019. Retrieved 3 January 2020.
- "By 2500 earth could be alien to humans". Scienmag: Latest Science and Health News. 14 October 2021. Archived from the original on 18 October 2021. Retrieved 18 October 2021.
- Lyon, Christopher; Saupe, Erin E.; Smith, Christopher J.; Hill, Daniel J.; Beckerman, Andrew P.; Stringer, Lindsay C.; Marchant, Robert; McKay, James; Burke, Ariane; O'Higgins, Paul; Dunhill, Alexander M.; Allen, Bethany J.; Riel-Salvatore, Julien; Aze, Tracy (2021). "Climate change research and action must look beyond 2100". Global Change Biology. 28 (2): 349–361. doi:10.1111/gcb.15871. ISSN 1365-2486. PMID 34558764. S2CID 237616583.
- Thomas R. Karl; Jerry M. Melillo; Thomas C. Peterson (eds.). "Global Climate Change". Global Climate Change Impacts in the United States (PDF). pp. 22–24. Archived (PDF) from the original on 15 November 2019. Retrieved 2 May 2013.
- "In-depth Q&A: The IPCC's sixth assessment report on climate science". Carbon Brief. 9 August 2021. Retrieved 12 February 2022.
- United Nations Environment Programme (UNEP) (November 2010), "Ch 2: Which emissions pathways are consistent with a 2 °C or a 1.5 °C temperature limit?: Sec 2.2 What determines long-term temperature?" (PDF), The Emissions Gap Report: Are the Copenhagen Accord pledges sufficient to limit global warming to 2 °C or 1.5 °C? A preliminary assessment (advance copy), UNEP, archived from the original (PDF) on 27 May 2011, p.28. This publication is also available in e-book format Archived 25 November 2010 at the Library of Congress Web Archives
- Collins, M.; Knutti, R.; Arblaster, J. M.; Dufresne, J.-L.; et al. (2013). "Chapter 12: Long-term Climate Change: Projections, Commitments and Irreversibility" (PDF). IPCC AR5 WG1 2013. p. 1104. Archived (PDF) from the original on 19 December 2019. Retrieved 3 January 2020.
- "Temperatures". Climate Action Tracker. 9 November 2021. Archived from the original on 26 January 2022.
- Irina Ivanova (2 June 2022). "California is rationing water amid its worst drought in 1,200 years". CBS News. Retrieved 2 June 2022.
- Trenberth, Kevin E. (2012). "Framing the way to relate climate extremes to climate change". Climatic Change. 115 (2): 283–290. doi:10.1007/s10584-012-0441-5. ISSN 0165-0009. S2CID 51990282.
- Trenberth, Kevin E.; Fasullo, John T.; Shepherd, Theodore G. (2015). "Attribution of climate extreme events". Nature Climate Change. 5 (8): 725–730. doi:10.1038/nclimate2657. ISSN 1758-678X.
- Rosenzweig; et al., "Chapter 1: Assessment of observed changes and responses in natural and managed systems", Contribution of Working Group II to the Fourth Assessment Report of the Intergovernmental Panel on Climate Change, 2007, Sec. 1.3.8.5 Summary of disasters and hazards, archived from the original on 23 December 2018, retrieved 28 December 2018, in IPCC AR4 WG2 2007.
- Effects of Global Warming Archived 6 December 2019 at the Wayback Machine, Live Science, 12 August 2017
- The science connecting extreme weather to climate change Archived 25 September 2019 at the Wayback Machine, Fact sheet: Union of Concerned Scientists, June 2018.
- Hausfather, Zeke (21 June 2017). "Study: Why troposphere warming differs between models and satellite data". Carbon Brief. Retrieved 19 November 2019.
- "Climate change: evidence and causes | Royal Society". royalsociety.org. Retrieved 19 November 2019.
- Trenberth, Ke (2011). "Changes in precipitation with climate change". Climate Research. 47 (1): 123–138. doi:10.3354/cr00953. ISSN 0936-577X.
- Douville, H., K. Raghavan, J. Renwick, R.P. Allan, P.A. Arias, M. Barlow, R. Cerezo-Mota, A. Cherchi, T.Y. Gan, J. Gergis, D. Jiang, A. Khan, W. Pokam Mba, D. Rosenfeld, J. Tierney, and O. Zolina, 2021: Chapter 8: Water Cycle Changes. In Climate Change 2021: The Physical Science Basis. Contribution of Working Group I to the Sixth Assessment Report of the Intergovernmental Panel on Climate Change [Masson-Delmotte, V., P. Zhai, A. Pirani, S.L. Connors, C. Péan, S. Berger, N. Caud, Y. Chen, L. Goldfarb, M.I. Gomis, M. Huang, K. Leitzell, E. Lonnoy, J.B.R. Matthews, T.K. Maycock, T. Waterfield, O. Yelekçi, R. Yu, and B. Zhou (eds.)]. Cambridge University Press, Cambridge, United Kingdom and New York, NY, USA, pp. 1055–1210, doi:10.1017/9781009157896.010
- Cheng, Lijing; Trenberth, Kevin E.; Gruber, Nicolas; Abraham, John P.; Fasullo, John T.; Li, Guancheng; Mann, Michael E.; Zhao, Xuanming; Zhu, Jiang (2020). "Improved Estimates of Changes in Upper Ocean Salinity and the Hydrological Cycle". Journal of Climate. 33 (23): 10357–10381. doi:10.1175/JCLI-D-20-0366.1. ISSN 0894-8755. S2CID 225289177.
- "Summary for policymakers", In IPCC SREX 2012, p. 8, archived from the original on 27 June 2019, retrieved 17 December 2012
- IPCC, Synthesis Report Summary for Policymakers Archived 9 March 2013 at the Wayback Machine, Section 3: Projected climate change and its impacts Archived 20 November 2017 at the Wayback Machine, in IPCC AR4 SYR 2007.
- NOAA (February 2007). "Will the wet get wetter and the dry drier?" (PDF). GFDL Climate Modeling Research Highlights. 1 (5): 1. Archived from the original (PDF) on 26 February 2013.
- Various authors (14 November 2014). "Projected increase in lightning strikes in the United States due to global warming". Science. doi:10.1126/science.1259100. Retrieved 29 October 2022.
{{cite web}}
: CS1 maint: uses authors parameter (link) - IPCC, 2021: Summary for Policymakers. In: Climate Change 2021: The Physical Science Basis. Contribution of Working Group I to the Sixth Assessment Report of the Intergovernmental Panel on Climate Change [Masson-Delmotte, V., P. Zhai, A. Pirani, S.L. Connors, C. Péan, S. Berger, N. Caud, Y. Chen, L. Goldfarb, M.I. Gomis, M. Huang, K. Leitzell, E. Lonnoy, J.B.R. Matthews, T.K. Maycock, T. Waterfield, O. Yelekçi, R. Yu, and B. Zhou (eds.)]. Cambridge University Press, Cambridge, United Kingdom and New York, NY, USA, pp. 3−32, doi:10.1017/9781009157896.001
- Rousi, Efi; Kornhuber, Kai; Beobide-Arsuaga, Goratz; Luo, Fei; Coumou, Dim (4 July 2022). "Accelerated western European heatwave trends linked to more-persistent double jets over Eurasia". Nature Communications. 13 (1): 3851. Bibcode:2022NatCo..13.3851R. doi:10.1038/s41467-022-31432-y. ISSN 2041-1723. PMC 9253148. PMID 35788585.
- News report: Fountain, Henry (18 July 2022). "Why Europe Is Becoming a Heat Wave Hot Spot". The New York Times. Retrieved 21 August 2022.
- Global Warming Makes Heat Waves More Likely, Study Finds Archived 7 August 2018 at the Wayback Machine 10 July 2012 The New York Times
- Hansen, J; Sato, M; Ruedy, R (2012). "Perception of climate change". Proceedings of the National Academy of Sciences. 109 (37): E2415–23. Bibcode:2012PNAS..109E2415H. doi:10.1073/pnas.1205276109. PMC 3443154. PMID 22869707.
- Heat wave: meteorology Archived 15 February 2020 at the Wayback Machine. Encyclopedia Britannica. Retrieved 1 April 2019.
- "Heat Waves: The Details". Climate Communication. Archived from the original on 12 July 2018. Retrieved 16 August 2018.
- Coumou, D.; Robinson, A.; Rahmstorf, S. (2013). "Global increase in record-breaking monthly-mean temperatures". Climatic Change. 118 (3–4): 771. Bibcode:2013ClCh..118..771C. doi:10.1007/s10584-012-0668-1. S2CID 121209624.
- Zhang, Yi; Held, Isaac; Fueglistaler, Stephan (8 March 2021). "Projections of tropical heat stress constrained by atmospheric dynamics". Nature Geoscience. 14 (3): 133–137. Bibcode:2021NatGe..14..133Z. doi:10.1038/s41561-021-00695-3. ISSN 1752-0908. S2CID 232146008.
- Milman, Oliver (8 March 2021). "Global heating pushes tropical regions towards limits of human livability". The Guardian. Retrieved 22 July 2022.
- IPCC (2013), Table SPM.1, in Summary for Policymakers, p. 5 (archived PDF), in IPCC AR5 WG1 2013
- Stocker, T.F., et al. (2013), Temperature Extremes, Heat Waves and Warm Spells, in: TFE.9, in: Technical Summary, p. 111 (archived PDF), in IPCC AR5 WG1 2013
- NOAA (16 February 2022). "Understanding the Arctic polar vortex". www.climate.gov. Retrieved 19 February 2022.
- "How global warming can cause Europe's harsh winter weather". Deutsche Welle. 11 February 2021. Retrieved 15 December 2021.
- "Climate change: Arctic warming linked to colder winters". BBC News. 2 September 2021. Archived from the original on 20 October 2021. Retrieved 20 October 2021.
- Cohen, Judah; Agel, Laurie; Barlow, Mathew; Garfinkel, Chaim I.; White, Ian (3 September 2021). "Linking Arctic variability and change with extreme winter weather in the United States". Science. 373 (6559): 1116–1121. Bibcode:2021Sci...373.1116C. doi:10.1126/science.abi9167. PMID 34516838. S2CID 237402139.
- Douglas, Erin (14 December 2021). "Winters get warmer with climate change. So what explains Texas' cold snap in February?". The Texas Tribune. Retrieved 15 December 2021.
- Hansen, James; Sato, Makiko; Ruedy, Reto; et al. (July 2012). "The New Climate Dice: Public Perception of Climate Change" (PDF). New York, USA: Dr James E. Hansen, Columbia University. pp. 3–4.
- Knutson, Thomas; Camargo, Suzana J.; Chan, Johnny C. L.; Emanuel, Kerry; Ho, Chang-Hoi; Kossin, James; Mohapatra, Mrutyunjay; Satoh, Masaki; Sugi, Masato; Walsh, Kevin; Wu, Liguang (6 August 2019). "Tropical Cyclones and Climate Change Assessment: Part II. Projected Response to Anthropogenic Warming". Bulletin of the American Meteorological Society. 101 (3): BAMS–D–18–0194.1. doi:10.1175/BAMS-D-18-0194.1. ISSN 0003-0007.
- "Major tropical cyclones have become '15% more likely' over past 40 years". Carbon Brief. 18 May 2020. Archived from the original on 8 August 2020. Retrieved 31 August 2020.
- Kossin, James P.; Knapp, Kenneth R.; Olander, Timothy L.; Velden, Christopher S. (18 May 2020). "Global increase in major tropical cyclone exceedance probability over the past four decades" (PDF). Proceedings of the National Academy of Sciences. 117 (22): 11975–11980. Bibcode:2020PNAS..11711975K. doi:10.1073/pnas.1920849117. ISSN 0027-8424. PMC 7275711. PMID 32424081. Archived (PDF) from the original on 19 November 2020. Retrieved 6 October 2020.
- Collins, M.; Sutherland, M.; Bouwer, L.; Cheong, S.-M.; et al. (2019). "Chapter 6: Extremes, Abrupt Changes and Managing Risks" (PDF). IPCC Special Report on the Ocean and Cryosphere in a Changing Climate. p. 602. Archived (PDF) from the original on 20 December 2019. Retrieved 6 October 2020.
- NASEM (2016). Attribution of Extreme Weather Events in the Context of Climate Change. Washington, D.C.: The National Academies Press. ISBN 978-0-309-38094-2. Retrieved 3 September 2021.
- Hu, Jane (19 December 2019). "The Decade of Attribution Science". Slate. Retrieved 3 September 2021.
- "The Science Connecting Extreme Weather to Climate Change". Union of Concerned Scientists. Retrieved 3 September 2021.
- Joyce, Christopher (10 December 2018). "Why Scientists Are Talking About Attribution Science And What It Is". NPR. Retrieved 3 September 2021.
- Zeng, Zubin (25 August 2021). "Is climate change to blame for extreme weather events? Attribution science says yes, for some – here's how it works". The Conversation. Retrieved 3 September 2021.
- US Department of Commerce, National Oceanic and Atmospheric Administration. "What is high tide flooding?". oceanservice.noaa.gov. Archived from the original on 16 October 2020. Retrieved 12 October 2020.
- Pörtner, Hans-O.; Roberts, Debra; Adam, Helen; Adler, Caroline; et al. "Summary for Policymakers" (PDF). Climate Change 2022: Impacts, Adaptation and Vulnerability. In Press. ¶SPM.B.4.5.
- Hirabayashi, Yukiko; Mahendran, Roobavannan; Koirala, Sujan; Konoshima, Lisako; Yamazaki, Dai; Watanabe, Satoshi; Kim, Hyungjun; Kanae, Shinjiro (2013). "Global flood risk under climate change". Nature Climate Change. 3 (9): 816–821. Bibcode:2013NatCC...3..816H. doi:10.1038/nclimate1911. ISSN 1758-6798.
- Hosseinzadehtalaei, Parisa; Tabari, Hossein; Willems, Patrick (November 2020). "Climate change impact on short-duration extreme precipitation and intensity–duration–frequency curves over Europe". Journal of Hydrology. 590: 125249. Bibcode:2020JHyd..59025249H. doi:10.1016/j.jhydrol.2020.125249. S2CID 224947610.
- Trenberth, Kevin E.; Dai, Aiguo; van der Schrier, Gerard; Jones, Philip D.; Barichivich, Jonathan; Briffa, Keith R.; Sheffield, Justin (2014). "Global warming and changes in drought" (PDF). Nature Climate Change. 4 (1): 17–22. doi:10.1038/nclimate2067. ISSN 1758-678X.
- Mukherjee, Sourav; Mishra, Ashok; Trenberth, Kevin E. (23 April 2018). "Climate Change and Drought: a Perspective on Drought Indices". Current Climate Change Reports. 4 (2): 145–163. doi:10.1007/s40641-018-0098-x. ISSN 2198-6061. S2CID 134811844.
- Mishra, A. K.; Singh, V. P. (2011). "Drought modeling – A review". Journal of Hydrology. 403 (1–2): 157–175. Bibcode:2011JHyd..403..157M. doi:10.1016/j.jhydrol.2011.03.049.
- Daniel Tsegai, Miriam Medel, Patrick Augenstein, Zhuojing Huang (2022) Drought in Numbers 2022 - restoration for readiness and resilience, United Nations Convention to Combat Desertification (UNCCD)
- IPCC SRCCL 2019, pp. 7, 8
- IPCC SRCCL Summary for Policymakers 2019, p. 7,8
- "Wildfire acres burned in the United States". OurWorldInData. 2021. Archived from the original on 12 October 2021. Data published by National Interagency Coordination Center; National Interagency Fire Center. (archive of NIFC data)
- Jones, Matthew; Smith, Adam; Betts, Richard; Canadell, Josep; Prentice, Collin; Le Quéré, Corrine. "Climate Change Increases the Risk of Wildfires". ScienceBrief. Retrieved 16 February 2022.
- Dunne, Daisy (14 July 2020). "Explainer: How climate change is affecting wildfires around the world". Carbon Brief. Retrieved 17 February 2022.
- State of the Climate in 2009, as appearing in the July 2010 issue (Vol. 91) of the Bulletin of the American Meteorological Society (BAMS). Supplemental and Summary Materials: Report at a Glance: Highlights (PDF). Website of the US National Oceanic and Atmospheric Administration: National Climatic Data Center. July 2010. Archived from the original (PDF) on 22 February 2011. Retrieved 6 June 2011.
- "NOAA: NESDIS: NCDC: Frequently Asked Questions: How do we know the Earth's climate is warming?". NOAA. 10 March 2010.
- Cheng, Lijing; Trenberth, Kevin E.; Gruber, Nicolas; Abraham, John P.; Fasullo, John T.; Li, Guancheng; Mann, Michael E.; Zhao, Xuanming; Zhu, Jiang (2020). "Improved Estimates of Changes in Upper Ocean Salinity and the Hydrological Cycle". Journal of Climate. 33 (23): 10357–10381. Bibcode:2020JCli...3310357C. doi:10.1175/jcli-d-20-0366.1. ISSN 0894-8755.
- IPCC, 2019: Summary for Policymakers. In: IPCC Special Report on the Ocean and Cryosphere in a Changing Climate [H.-O. Pörtner, D.C. Roberts, V. Masson-Delmotte, P. Zhai, M. Tignor, E. Poloczanska, K. Mintenbeck, A. Alegría, M. Nicolai, A. Okem, J. Petzold, B. Rama, N.M. Weyer (eds.)]. Cambridge University Press, Cambridge, UK and New York, NY, USA. https://doi.org/10.1017/9781009157964.001.
- Cheng, Lijing; Abraham, John; Hausfather, Zeke; Trenberth, Kevin E. (2019). "How fast are the oceans warming?". Science. 363 (6423): 128–129. Bibcode:2019Sci...363..128C. doi:10.1126/science.aav7619. ISSN 0036-8075. PMID 30630919. S2CID 57825894.
- Doney, Scott C.; Busch, D. Shallin; Cooley, Sarah R.; Kroeker, Kristy J. (17 October 2020). "The Impacts of Ocean Acidification on Marine Ecosystems and Reliant Human Communities". Annual Review of Environment and Resources. 45 (1): 83–112. doi:10.1146/annurev-environ-012320-083019. ISSN 1543-5938.
Text was copied from this source, which is available under a Creative Commons Attribution 4.0 International License
- Bindoff, N.L., W.W.L. Cheung, J.G. Kairo, J. Arístegui, V.A. Guinder, R. Hallberg, N. Hilmi, N. Jiao, M.S. Karim, L. Levin, S. O’Donoghue, S.R. Purca Cuicapusa, B. Rinkevich, T. Suga, A. Tagliabue, and P. Williamson, 2019: Chapter 5: Changing Ocean, Marine Ecosystems, and Dependent Communities. In: IPCC Special Report on the Ocean and Cryosphere in a Changing Climate [H.-O. Pörtner, D.C. Roberts, V. Masson-Delmotte, P. Zhai, M. Tignor, E. Poloczanska, K. Mintenbeck, A. Alegría, M. Nicolai, A. Okem, J. Petzold, B. Rama, N.M. Weyer (eds.)]. In press.
- Lee, Tsz-Cheung; Knutson, Thomas R.; Nakaegawa, Toshiyuki; Ying, Ming; Cha, Eun Jeong (March 2020). "Third assessment on impacts of climate change on tropical cyclones in the Typhoon Committee Region – Part I: Observed changes, detection and attribution". Tropical Cyclone Research and Review. 9 (1): 1–22. doi:10.1016/j.tcrr.2020.03.001. ISSN 2225-6032.
- Freedman, Andrew (29 September 2020). "Mixing of the planet's ocean waters is slowing down, speeding up global warming, study finds". The Washington Post. ISSN 0190-8286. Archived from the original on 15 October 2020. Retrieved 12 October 2020.
- Chester, R.; Jickells, Tim (2012). "Chapter 9: Nutrients oxygen organic carbon and the carbon cycle in seawater". Marine geochemistry (3rd ed.). Chichester, West Sussex, UK: Wiley/Blackwell. ISBN 978-1-118-34909-0. OCLC 781078031.
- Riebeek, H.. design by R. Simmon (9 May 2006). "Paleoclimatology: Explaining the Evidence: Explaining Rapid Climate Change: Tales from the Ice". NASA Earth Observatory. Archived from the original on 27 January 2019. Retrieved 16 October 2011.
- Slater, Thomas; Lawrence, Isobel R.; Otosaka, Inès N.; Shepherd, Andrew; et al. (25 January 2021). "Review article: Earth's ice imbalance". The Cryosphere. 15 (1): 233–246. Bibcode:2021TCry...15..233S. doi:10.5194/tc-15-233-2021. ISSN 1994-0416. S2CID 234098716. Archived from the original on 26 January 2021. Retrieved 26 January 2021. Fig. 4.
- Getting to Know the Cryosphere Archived 15 December 2019 at the Wayback Machine, Earth Labs
- IPCC (2019). "Technical Summary" (PDF). In Pörtner, H.-O.; Roberts, D.C.; Masson-Delmotte, V.; Zhai, P.; et al. (eds.). IPCC SROCC 2019. pp. 39–69. Archived (PDF) from the original on 18 October 2020. Retrieved 28 August 2020.
- Mass Balance of Mountain Glaciers in 2011, archived from the original on 14 June 2013, retrieved 20 March 2013, in Kennedy 2012
- Glavovic, B.; Oppenheimer, M.; Abd-Elgawad, A.; Cai, R.; et al. (2019). "Chapter 4: Sea Level Rise and Implications for Low Lying Islands, Coasts and Communities" (PDF). IPCC SROCC 2019. p. 234. Archived (PDF) from the original on 26 November 2019. Retrieved 21 November 2019.
- Fox-Kemper, B., H.T. Hewitt, C. Xiao, G. Aðalgeirsdóttir, S.S. Drijfhout, T.L. Edwards, N.R. Golledge, M. Hemer, R.E. Kopp, G. Krinner, A. Mix, D. Notz, S. Nowicki, I.S. Nurhati, L. Ruiz, J.-B. Sallée, A.B.A. Slangen, and Y. Yu, 2021: Chapter 9: Ocean, Cryosphere and Sea Level Change. In Climate Change 2021: The Physical Science Basis. Contribution of Working Group I to the Sixth Assessment Report of the Intergovernmental Panel on Climate Change [Masson-Delmotte, V., P. Zhai, A. Pirani, S.L. Connors, C. Péan, S. Berger, N. Caud, Y. Chen, L. Goldfarb, M.I. Gomis, M. Huang, K. Leitzell, E. Lonnoy, J.B.R. Matthews, T.K. Maycock, T. Waterfield, O. Yelekçi, R. Yu, and B. Zhou (eds.)]. Cambridge University Press, Cambridge, United Kingdom and New York, NY, USA, pp. 1211–1362, doi:10.1017/9781009157896.011.
- Greene, Chad A.; Gardner, Alex S.; Schlegel, Nicole-Jeanne; Fraser, Alexander D. (10 August 2022). "Antarctic calving loss rivals ice-shelf thinning". Nature. 609 (7929): 948–953. doi:10.1038/s41586-022-05037-w. ISSN 1476-4687. PMID 35948639. S2CID 251495070.
- Nilsson, Johan; Gardner, Alex S.; Paolo, Fernando S. (10 August 2022). "Elevation change of the Antarctic Ice Sheet: 1985 to 2020". Earth System Science Data. 14 (8): 3573–3598. doi:10.5194/essd-14-3573-2022. ISSN 1866-3508.
- Collins, M.; Sutherland, M.; Bouwer, L.; Cheong, S. M.; Frölicher, T.; et al. (2019). "Chapter 6: Extremes, Abrupt Changes and Managing Risks" (PDF). IPCC SROCC 2019. pp. 595–596. Archived (PDF) from the original on 20 December 2019. Retrieved 21 December 2019.
- Meredith, M.; Sommerkorn, M.; Cassotta, S.; Derksen, C.; Ekaykin, A.; et al. (2019). "Chapter 3: Polar Regions" (PDF). IPCC SROCC 2019. pp. 244–246. Archived (PDF) from the original on 19 December 2019. Retrieved 21 December 2019.
- Glavovic, B.; Oppenheimer, M.; Abd-Elgawad, A.; Cai, R.; et al. (2019). "Chapter 4: Sea Level Rise and Implications for Low Lying Islands, Coasts and Communities" (PDF). IPCC SROCC 2019. p. 363. Archived (PDF) from the original on 26 November 2019. Retrieved 21 November 2019.
- Glavovic, B.; Oppenheimer, M.; Abd-Elgawad, A.; Cai, R.; Cifuentes-Jara, M.; et al. (2019). "Chapter 4: Sea Level Rise and Implications for Low Lying Islands, Coasts and Communities" (PDF). IPCC SROCC 2019. p. 362. Archived (PDF) from the original on 26 November 2019. Retrieved 21 November 2019.
- "Thermodynamics: Albedo | National Snow and Ice Data Center". nsidc.org. Archived from the original on 11 October 2017. Retrieved 14 October 2020.
- "Arctic Report Card 2012". NOAA. Retrieved 8 May 2013.
- Impacts of a melting cryosphere ice loss around the world Archived 15 December 2019 at the Wayback Machine, Carbon Brief, 9 June 2011
- 2011 Arctic Sea Ice Minimum, archived from the original on 14 June 2013, retrieved 20 March 2013, in Kennedy 2012
- Kwok, R. (12 October 2018). "Arctic sea ice thickness, volume, and multiyear ice coverage: losses and coupled variability (1958–2018)". Environmental Research Letters. 13 (10): 105005. doi:10.1088/1748-9326/aae3ec. ISSN 1748-9326.
- IPCC (2018). "Summary for Policymakers" (PDF). IPCC SR15 2018. p. 8. Archived (PDF) from the original on 23 July 2021. Retrieved 19 December 2019.
- Watts, Jonathan (27 February 2018). "Arctic warming: scientists alarmed by 'crazy' temperature rises". The Guardian.
- Romanovsky, Vladimir. "How rapidly is permafrost changing and what are the impacts of these changes?". NOAA. Retrieved 6 December 2007.
- Paton Walsh, Nick (10 June 2005). "Shrinking lakes of Siberia blamed on global warming". The Guardian.
- Fox-Kemper, B., H.T. Hewitt, C. Xiao, G. Aðalgeirsdóttir, S.S. Drijfhout, T.L. Edwards, N.R. Golledge, M. Hemer, R.E. Kopp, G. Krinner, A. Mix, D. Notz, S. Nowicki, I.S. Nurhati, L. Ruiz, J.-B. Sallée, A.B.A. Slangen, and Y. Yu, 2021: Chapter 9: Ocean, Cryosphere and Sea Level Change. In Climate Change 2021: The Physical Science Basis. Contribution of Working Group I to the Sixth Assessment Report of the Intergovernmental Panel on Climate Change [Masson-Delmotte, V., P. Zhai, A. Pirani, S.L. Connors, C. Péan, S. Berger, N. Caud, Y. Chen, L. Goldfarb, M.I. Gomis, M. Huang, K. Leitzell, E. Lonnoy, J.B.R. Matthews, T.K. Maycock, T. Waterfield, O. Yelekçi, R. Yu, and B. Zhou (eds.)]. Cambridge University Press, Cambridge, United Kingdom and New York, NY, USA, pp. 1211–1362, doi:10.1017/9781009157896.011.
- Koven, Charles D.; Riley, William J.; Stern, Alex (1 October 2012). "Analysis of Permafrost Thermal Dynamics and Response to Climate Change in the CMIP5 Earth System Models". Journal of Climate. 26 (6): 1877–1900. doi:10.1175/JCLI-D-12-00228.1. ISSN 0894-8755. OSTI 1172703.
- Armstrong McKay, David I.; Staal, Arie; Abrams, Jesse F.; Winkelmann, Ricarda; Sakschewski, Boris; Loriani, Sina; Fetzer, Ingo; Cornell, Sarah E.; Rockström, Johan; Lenton, Timothy M. (9 September 2022). "Exceeding 1.5°C global warming could trigger multiple climate tipping points". Science. 377 (6611): eabn7950. doi:10.1126/science.abn7950. ISSN 0036-8075.
- Nelson, F. E.; Anisimov, O. A.; Shiklomanov, N. I. (1 July 2002). "Climate Change and Hazard Zonation in the Circum-Arctic Permafrost Regions". Natural Hazards. 26 (3): 203–225. doi:10.1023/A:1015612918401. ISSN 1573-0840. S2CID 35672358.
- Barry, Roger Graham; Gan, Thian-Yew (2021). The global cryosphere past, present and future (Second revised ed.). Cambridge, United Kingdom. ISBN 978-1-108-48755-9. OCLC 1256406954.
- The Natural Fix?: The Role of Ecosystems in Climate Mitigation UNEP 2009 page. 20, 55
- Díaz, S.; et al. (2019). Summary for policymakers of the global assessment report on biodiversity and ecosystem services of the Intergovernmental Science-Policy Platform on Biodiversity and Ecosystem Services (PDF). Bonn, Germany: ISBES secretariat. p. 12. Archived (PDF) from the original on 23 July 2021. Retrieved 28 December 2019.
- Díaz, S.; et al. (2019). Summary for policymakers of the global assessment report on biodiversity and ecosystem services of the Intergovernmental Science-Policy Platform on Biodiversity and Ecosystem Services (PDF). Bonn, Germany: ISBES secretariat. p. 16. Archived (PDF) from the original on 23 July 2021. Retrieved 28 December 2019.
- Van Riper, Charles. (2014) Projecting Climate Effects on Birds and Reptiles of the Southwestern United States. Archived 23 July 2021 at the Wayback Machine Reston, Va.: U.S. Department of the Interior, U.S. Geological Survey.
- McElwee, Pamela (1 November 2021). "Climate Change and Biodiversity Loss: Two Sides of the Same Coin". Current History. 120 (829): 295–300. doi:10.1525/curh.2021.120.829.295. ISSN 0011-3530. S2CID 240056779.
- Meyer, Andreas L. S.; Bentley, Joanne; Odoulami, Romaric C.; Pigot, Alex L.; Trisos, Christopher H. (15 August 2022). "Risks to biodiversity from temperature overshoot pathways". Philosophical Transactions of the Royal Society B: Biological Sciences. 377 (1857): 20210394. doi:10.1098/rstb.2021.0394. PMC 9234811. PMID 35757884.
- Rosenzweig, C. (December 2008). "Science Briefs: Warming Climate is Changing Life on Global Scale". Website of the US National Aeronautics and Space Administration, Goddard Institute for Space Studies. Archived from the original on 4 April 2009. Retrieved 8 July 2011.
- February 23; Denchak, 2017 Melissa. "Global Climate Change: What You Need to Know". NRDC. Archived from the original on 19 October 2020. Retrieved 11 October 2020.
- Fischlin; et al., "Chapter 4: Ecosystems, their properties, goods and services", Contribution of Working Group II to the Fourth Assessment Report of the Intergovernmental Panel on Climate Change, 2007, Sec. 4.4.11 Global synthesis including impacts on biodiversity, archived from the original on 28 December 2018, retrieved 28 December 2018, in IPCC AR4 WG2 2007.
- Settele, J.; Scholes, R.; Betts, R.; Bunn, S.; et al. (2014). "Chapter 4: Terrestrial and Inland Water Systems" (PDF). IPCC AR5 WG2 A 2014. p. 275. Archived (PDF) from the original on 19 December 2019. Retrieved 2 January 2020.
- Butler, Rhett A. (31 March 2021). "Global forest loss increases in 2020". Mongabay. Archived from the original on 1 April 2021. ● Mongabay graphing WRI data from "Forest Loss / How much tree cover is lost globally each year?". research.WRI.org. World Resources Institute — Global Forest Review. January 2021. Archived from the original on 10 March 2021.
- Lovejoy, Thomas E.; Nobre, Carlos (2019). "Amazon tipping point: Last chance for action". Science Advances. 5 (12): eaba2949. Bibcode:2019SciA....5A2949L. doi:10.1126/sciadv.aba2949. PMC 6989302. PMID 32064324.
- "Ecosystems the size of Amazon 'can collapse within decades'". The Guardian. 10 March 2020. Archived from the original on 12 April 2020. Retrieved 13 April 2020.
- Cooper, Gregory S.; Willcock, Simon; Dearing, John A. (10 March 2020). "Regime shifts occur disproportionately faster in larger ecosystems". Nature Communications. 11 (1): 1175. Bibcode:2020NatCo..11.1175C. doi:10.1038/s41467-020-15029-x. ISSN 2041-1723. PMC 7064493. PMID 32157098.
- Smale, Dan A.; Wernberg, Thomas; Oliver, Eric C. J.; Thomsen, Mads; Harvey, Ben P.; Straub, Sandra C.; Burrows, Michael T.; Alexander, Lisa V.; Benthuysen, Jessica A.; Donat, Markus G.; Feng, Ming (2019). "Marine heatwaves threaten global biodiversity and the provision of ecosystem services". Nature Climate Change. 9 (4): 306–312. Bibcode:2019NatCC...9..306S. doi:10.1038/s41558-019-0412-1. ISSN 1758-6798. S2CID 91471054. Archived from the original on 29 July 2020. Retrieved 30 August 2020.
- Bindoff, N. L.; Cheung, W. W. L.; Kairo, J. G.; Arístegui, J.; et al. (2019). "Chapter 5: Changing Ocean, Marine Ecosystems, and Dependent Communities" (PDF). IPCC SROCC 2019. p. 451. Archived (PDF) from the original on 28 May 2020. Retrieved 24 May 2020.
- Riebesell, Ulf; Körtzinger, Arne; Oschlies, Andreas (2009). "Sensitivities of marine carbon fluxes to ocean change". PNAS. 106 (49): 20602–20609. doi:10.1073/pnas.0813291106. PMC 2791567. PMID 19995981.
- Bindoff, N. L.; Cheung, W. W. L.; Kairo, J. G.; Arístegui, J.; Guinder, V. A.; et al. (2019). "Chapter 5: Changing Ocean, Marine Ecosystems, and Dependent Communities" (PDF). IPCC SROCC 2019. p. 450. Archived (PDF) from the original on 19 December 2019. Retrieved 21 November 2019.
- Hoegh-Guldberg, O.; Jacob, D.; Taylor, M.; Bindi, M.; et al. (2018). "Chapter 3: Impacts of 1.5ºC Global Warming on Natural and Human Systems" (PDF). IPCC SR15 2018. p. 266. Archived (PDF) from the original on 15 November 2019. Retrieved 15 December 2019.
- Kopp, R. E.; Hayhoe, K.; Easterling, D.R.; Hall, T.; et al. (2017). "Chapter 15: Potential Surprises: Compound Extremes and Tipping Elements". In USGCRP 2017 . US National Climate Assessment. p. 411. Archived from the original on 20 August 2018.
- Kopp, R. E.; Hayhoe, K.; Easterling, D.R.; Hall, T.; et al. (2017). "Chapter 15: Potential Surprises: Compound Extremes and Tipping Elements". In USGCRP 2017 . US National Climate Assessment. p. 417. Archived from the original on 20 August 2018.
- Carrington, Damian (27 November 2019). "Climate emergency: world 'may have crossed tipping points'". The Guardian. Archived from the original on 4 January 2020. Retrieved 4 January 2020.
- Hoegh-Guldberg, O.; Jacob, D.; Taylor, M.; Bindi, M.; et al. (2018). "Chapter 3: Impacts of 1.5ºC Global Warming on Natural and Human Systems" (PDF). IPCC SR15 2018. pp. 254, 258. Archived (PDF) from the original on 15 November 2019. Retrieved 15 December 2019.
- Lontzek, Thomas S.; Cai, Yongyang; Judd, Kenneth L.; Lenton, Timothy M. (2015). "Stochastic integrated assessment of climate tipping points indicates the need for strict climate policy". Nature Climate Change. 5 (5): 441–444. Bibcode:2015NatCC...5..441L. doi:10.1038/nclimate2570. hdl:10871/35041. ISSN 1758-6798. S2CID 84760180. Archived from the original on 20 December 2019. Retrieved 23 December 2019.
- Lenton, Timothy M.; Rockström, Johan; Gaffney, Owen; Rahmstorf, Stefan; Richardson, Katherine; Steffen, Will; Schellnhuber, Hans Joachim (2019). "Climate tipping points — too risky to bet against". Nature. 575 (7784): 592–595. Bibcode:2019Natur.575..592L. doi:10.1038/d41586-019-03595-0. PMID 31776487.
- Carrington, Damian (3 June 2021). "Climate tipping points could topple like dominoes, warn scientists". The Guardian. Archived from the original on 7 June 2021. Retrieved 8 June 2021.
- C. Rocha, Juan; Peterson, Garry; Bodin, Örjan; Levin, Simon (21 December 2018). "Cascading regime shifts within and across scales". Science. 362 (6421): 1379–1383. Bibcode:2018Sci...362.1379R. doi:10.1126/science.aat7850. PMID 30573623. S2CID 56582186.
- Watts, Jonathan (20 December 2018). "Risks of 'domino effect' of tipping points greater than thought, study says". The Guardian. Archived from the original on 7 February 2019. Retrieved 24 December 2018.
- Schneider; et al., "Chapter 19: Assessing key vulnerabilities and the risk from climate change", In IPCC AR4 WG2 2007, Sec.19.2 Criteria for selecting 'key' vulnerabilities: Persistence and reversibility, archived from the original on 23 December 2018, retrieved 28 December 2018
- Sabūnas, Audrius; Miyashita, Takuya; Fukui, Nobuki; Shimura, Tomoya; Mori, Nobuhito (10 November 2021). "Impact Assessment of Storm Surge and Climate Change-Enhanced Sea Level Rise on Atoll Nations: A Case Study of the Tarawa Atoll, Kiribati". Frontiers in Built Environment. 7. doi:10.3389/fbuil.2021.752599.
- Barnett, J; WN Adger (2003). "Climate dangers and atoll countries" (PDF). Climatic Change. Kluwer Academic Publishers. 61 (3): 321–337. doi:10.1023/b:clim.0000004559.08755.88. S2CID 55644531. Archived from the original (PDF) on 31 October 2012. Retrieved 31 October 2011. This paper was published in 2001 as Tyndall Centre Working Paper 9 Archived 16 June 2012 at the Wayback Machine
- Kemp, Luke; Xu, Chi; Depledge, Joanna; Ebi, Kristie L.; Gibbins, Goodwin; Kohler, Timothy A.; Rockström, Johan; Scheffer, Marten; Schellnhuber, Hans Joachim; Steffen, Will; Lenton, Timothy M. (23 August 2022). "Climate Endgame: Exploring catastrophic climate change scenarios". Proceedings of the National Academy of Sciences. 119 (34): e2108146119. Bibcode:2022PNAS..11908146K. doi:10.1073/pnas.2108146119. ISSN 0027-8424. PMC 9407216. PMID 35914185.
- "Human Health: Impacts, Adaptation, and Co-Benefits — IPCC". Archived from the original on 31 October 2020. Retrieved 11 October 2020.
- "Water and the global climate crisis: 10 things you should know". www.unicef.org. Archived from the original on 27 October 2021. Retrieved 27 October 2021.
- "WHO calls for urgent action to protect health from climate change – Sign the call". www.who.int. World Health Organization. 2015. Archived from the original on 8 October 2015. Retrieved 19 April 2020.
- Marina Romanello; et al. (2021). "The 2021 report of the Lancet Countdown on health and climate change: code red for a healthy future" (PDF). The Lancet. 398 (10311): 1619–1662. doi:10.1016/S0140-6736(21)01787-6. hdl:10278/3746207. ISSN 0140-6736. PMID 34687662. S2CID 239046862.
- McMichael, Anthony J; Woodruff, Rosalie E; Hales, Simon (March 2006). "Climate change and human health: present and future risks". The Lancet. 367 (9513): 859–869. doi:10.1016/s0140-6736(06)68079-3. ISSN 0140-6736. PMID 16530580. S2CID 11220212.
- Easterling; et al., "Chapter 5: Food, Fibre, and Forest Products", In IPCC AR4 WG2 2007, p. 282, archived from the original on 23 December 2018, retrieved 28 December 2018
- Ding, Y.; Hayes, M. J.; Widhalm, M. (2011). "Measuring economic impacts of drought: A review and discussion". Disaster Prevention and Management. 20 (4): 434–446. doi:10.1108/09653561111161752. Archived from the original on 23 July 2018. Retrieved 14 July 2019.
- Ndiritu, S. Wagura; Muricho, Geoffrey (2021). "Impact of climate change adaptation on food security: evidence from semi-arid lands, Kenya". Climatic Change. 167 (1–2): 24. doi:10.1007/s10584-021-03180-3. ISSN 0165-0009.
- IPCC SRCCL Summary for Policymakers 2019, p. 5.
- Mbow, C.; Rosenzweig, C.; Barioni, L. G.; Benton, T.; et al. (2019). "Chapter 5: Food Security" (PDF). IPCC SRCCL 2019. p. 442. Archived (PDF) from the original on 27 November 2019. Retrieved 24 December 2019.
- Vermeulen, Sonja J.; Campbell, Bruce M.; Ingram, John S.I. (21 November 2012). "Climate Change and Food Systems". Annual Review of Environment and Resources. 37 (1): 195–222. doi:10.1146/annurev-environ-020411-130608. ISSN 1543-5938. S2CID 28974132. Retrieved 11 July 2022.
- Carter, Colin; Cui, Xiaomeng; Ghanem, Dalia; Mérel, Pierre (5 October 2018). "Identifying the Economic Impacts of Climate Change on Agriculture". Annual Review of Resource Economics. 10 (1): 361–380. doi:10.1146/annurev-resource-100517-022938. ISSN 1941-1340. S2CID 158817046. Retrieved 11 July 2022.
- Chrobak, Ula; Zimmer, Katarina (22 June 2022). "Climate change is altering the chemistry of wine". Knowable Magazine. doi:10.1146/knowable-062222-1. Retrieved 11 July 2022.
- Bindoff, N. L.; Cheung, W. W. L.; Kairo, J. G.; Arístegui, J.; Guinder, V. A.; et al. (2019). "Chapter 5: Changing Ocean, Marine Ecosystems, and Dependent Communities" (PDF). IPCC SROCC 2019. p. 504. Archived (PDF) from the original on 19 December 2019. Retrieved 21 November 2019.
- Caretta, Martina Angela; Mukherji, Aditi; et al. "Chapter 4: Water" (PDF). Climate Change 2022: Impacts, Adaptation and Vulnerability. Contribution of Working Group II to the Sixth Assessment Report of the Intergovernmental Panel on Climate Change. Intergovernmental Panel on Climate Change. FAQ4.1.
- Jiménez Cisneros, B. E.; Oki, T.; Arnell, N. W.; Benito, G.; et al. (2014). "Chapter 3: Freshwater Resources" (PDF). IPCC AR5 WG2 A 2014. p. 251. Archived (PDF) from the original on 19 December 2019. Retrieved 26 December 2019.
- Caretta, M.A., A. Mukherji, M. Arfanuzzaman, R.A. Betts, A. Gelfan, Y. Hirabayashi, T.K. Lissner, J. Liu, E. Lopez Gunn, R. Morgan, S. Mwanga, and S. Supratid, 2022: water is about drinking water Water (Chapter 4). In: Climate Change 2022: Impacts, Adaptation, and Vulnerability. Contribution of Working Group II to the Sixth Assessment Report of the Intergovernmental Panel on Climate Change [H.-O. Pörtner, D.C. Roberts, M. Tignor, E.S. Poloczanska, K. Mintenbeck, A. Alegría, M. Craig, S. Langsdorf, S. Löschke, V. Möller, A. Okem, B. Rama (eds.)]. Cambridge University Press. In Press.
- Grey, David; Sadoff, Claudia W. (2007). "Sink or Swim? Water security for growth and development" (PDF). Water Policy. 9 (6): 545–571. doi:10.2166/wp.2007.021. ISSN 1366-7017.
- Sadoff, Claudia; Grey, David; Borgomeo, Edoardo (2020), "Water Security", Oxford Research Encyclopedia of Environmental Science, Oxford University Press, doi:10.1093/acrefore/9780199389414.013.609, ISBN 978-0-19-938941-4, retrieved 12 April 2022
- UN-Water (2013) Water Security & the Global Water Agenda - A UN-Water Analytical Brief, ISBN 978-92-808-6038-2, United Nations University
- Kompas, Tom; Pham, Van Ha; Che, Tuong Nhu (2018). "The Effects of Climate Change on GDP by Country and the Global Economic Gains From Complying With the Paris Climate Accord". Earth's Future. 6 (8): 1153–1173. Bibcode:2018EaFut...6.1153K. doi:10.1029/2018EF000922. ISSN 2328-4277.
- Bouwer, Laurens M. (2019), Mechler, Reinhard; Bouwer, Laurens M.; Schinko, Thomas; Surminski, Swenja (eds.), "Observed and Projected Impacts from Extreme Weather Events: Implications for Loss and Damage", Loss and Damage from Climate Change: Concepts, Methods and Policy Options, Climate Risk Management, Policy and Governance, Cham: Springer International Publishing, pp. 63–82, doi:10.1007/978-3-319-72026-5_3, ISBN 978-3-319-72026-5
- IPCC, Synthesis Report, Question 2, Sections 2.25 and 2.26, archived from the original on 5 March 2016, retrieved 21 June 2012, p. 55, IPCC TAR SYR 2001 .
- Diffenbaugh, Noah S.; Burke, Marshall (2019). "Global warming has increased global economic inequality". Proceedings of the National Academy of Sciences. 116 (20): 9808–9813. doi:10.1073/pnas.1816020116. ISSN 0027-8424. PMC 6525504. PMID 31010922.
- Begum, Rawshan Ara; Lempert, Robert; et al. "Chapter 1: Point of Departure and Key Concept" (PDF). Climate Change 2022: Impacts, Adaptation and Vulnerability. Contribution of Working Group II to the Sixth Assessment Report of the Intergovernmental Panel on Climate Change. Intergovernmental Panel on Climate Change. Section 1.3.2.1.
-
- IPCC (2014). "Summary for Policymakers" (PDF). IPCC AR5 WG2 A 2014. p. 12. Archived (PDF) from the original on 19 December 2019. Retrieved 15 February 2020.
- Hoegh-Guldberg, O.; Jacob, D.; Taylor, M.; Bindi, M.; et al. (2018). "Chapter 3: Impacts of 1.5 ºC Global Warming on Natural and Human Systems" (PDF). IPCC SR15 2018. p. 256. Archived (PDF) from the original on 15 November 2019. Retrieved 15 December 2019.
- Koning Beals, Rachel. "Global GDP will suffer at least a 3% hit by 2050 from unchecked climate change, say economists". MarketWatch. Archived from the original on 29 March 2020. Retrieved 29 March 2020.
- Dr. Frauke Urban and Dr. Tom Mitchell 2011. Climate change, disasters and electricity generation Archived 20 September 2012 at the Wayback Machine. London: Overseas Development Institute and Institute of Development Studies
- Nichols, Will; Clisby, Rory. "40% of Oil and Gas Reserves Threatened by Climate Change". Verisk Maplecroft. Retrieved 15 February 2022.
- Surminski, Swenja; Bouwer, Laurens M.; Linnerooth-Bayer, Joanne (2016). "How insurance can support climate resilience". Nature Climate Change. 6 (4): 333–334. Bibcode:2016NatCC...6..333S. doi:10.1038/nclimate2979. ISSN 1758-6798.
- Neslen, Arthur (21 March 2019). "Climate change could make insurance too expensive for most people – report". Theguardian.com. Retrieved 22 March 2019.
- Studies Show Climate Change Melting Permafrost Under Runways in Western Arctic Archived 27 September 2011 at the Wayback Machine Weber, Bob Airportbusiness.com October 2007
- O'Brien, Karen L; Leichenko, Robin M (1 October 2000). "Double exposure: assessing the impacts of climate change within the context of economic globalization". Global Environmental Change. 10 (3): 221–232. doi:10.1016/S0959-3780(00)00021-2. ISSN 0959-3780.
- Zhang, Li; Chen, Fu; Lei, Yongdeng (2020). "Climate change and shifts in cropping systems together exacerbate China's water scarcity". Environmental Research Letters. 15 (10): 104060. Bibcode:2020ERL....15j4060Z. doi:10.1088/1748-9326/abb1f2. S2CID 225127981.
- Cramer, Wolfgang; Guiot, Joël; Fader, Marianela; Garrabou, Joaquim; Gattuso, Jean-Pierre; Iglesias, Ana; Lange, Manfred A.; Lionello, Piero; Llasat, Maria Carmen; Paz, Shlomit; Peñuelas, Josep; Snoussi, Maria; Toreti, Andrea; Tsimplis, Michael N.; Xoplaki, Elena (November 2018). "Climate change and interconnected risks to sustainable development in the Mediterranean". Nature Climate Change. 8 (11): 972–980. Bibcode:2018NatCC...8..972C. doi:10.1038/s41558-018-0299-2. ISSN 1758-6798. S2CID 92556045.
- "MOTION FOR A RESOLUTION on the 2021 UN Climate Change Conference in Glasgow, UK (COP26)". www.europarl.europa.eu. Archived from the original on 29 October 2021. Retrieved 29 October 2021.
- Watts, Jonathan (5 May 2020). "One billion people will live in insufferable heat within 50 years – study". The Guardian. Archived from the original on 7 May 2020. Retrieved 7 May 2020.
- Xu, Chi; M. Lenton, Timothy; Svenning, Jens-Christian; Scheffer, Marten (26 May 2020). "Future of the human climate niche". Proceedings of the National Academy of Sciences of the United States of America. 117 (21): 11350–11355. Bibcode:2020PNAS..11711350X. doi:10.1073/pnas.1910114117. PMC 7260949. PMID 32366654.
- Ripple, William J.; Wolf, Christopher; Newsome, Thomas M.; Barnard, Phoebe; Moomaw, William R. (2020). "World Scientists' Warning of a Climate Emergency". BioScience. 70 (1): 8–12. doi:10.1093/biosci/biz088. ISSN 0006-3568. Archived from the original on 15 April 2021. Retrieved 25 September 2020.
- World Scientists' Warning of a Climate Emergency, William J Ripple, Christopher Wolf, Thomas M Newsome, Phoebe Barnard, William R Moomaw. BioScience, biz088, https://doi.org/10.1093/biosci/biz088. A correction has been published: BioScience, biz152 Archived 7 January 2020 at the Wayback Machine, https://doi.org/10.1093/biosci/biz152 Archived 28 February 2020 at the Wayback Machine
- Scientists Around the World Declare 'Climate Emergency' Archived 16 December 2019 at the Wayback Machine, Smithsonian Magazine, 5 November 2019
- Climate change could pose 'existential threat' by 2050: report Archived 27 January 2020 at the Wayback Machine, CNN, 5 June 2019.
- Climate tipping points — too risky to bet against Archived 5 February 2020 at the Wayback Machine, Nature, 27 November 2019.
- Greta Thunberg showed the world what it means to lead Archived 29 October 2021 at the Wayback Machine, The Guardian, 25 September 2019
- Kaczan, David J.; Orgill-Meyer, Jennifer (2020). "The impact of climate change on migration: a synthesis of recent empirical insights". Climatic Change. 158 (3): 281–300. Bibcode:2020ClCh..158..281K. doi:10.1007/s10584-019-02560-0. ISSN 1573-1480. S2CID 207988694.
- GRID Internal displacement in a changing climate (PDF). Internal Displacement Monitoring Center. 2021. pp. 42–53. Retrieved 24 May 2021.
- Niranjan, Ajit (21 May 2021). "Extreme Weather Displaces Record Numbers of People as Temperatures Rise". Ecowatch. Retrieved 24 May 2021.
- Environmental Migrants: Up To 1 Billion By 2050, Centro Euro-Mediterraneo sui Cambiamenti Climactici (CMCC)
- 143 Million People May Soon Become Climate Migrants Archived 19 December 2019 at the Wayback Machine, National Geographic, 19 March 2018
- Kumari Rigaud, Kanta; de Sherbinin, Alex; Jones, Bryan; et al. (2018). Groundswell: preparing for internal climate migration (PDF). Washington DC: The World Bank. p. xxi. Archived (PDF) from the original on 2 January 2020. Retrieved 29 December 2019.
- "Addressing Climate Change and Migration in Asia and the Pacific: Final Report" (PDF). Asian Development Bank. 6 April 2015. Archived from the original (PDF) on 6 April 2015. Retrieved 22 March 2019.
- Cheshirkov, Boris (10 December 2021). "Climate change fuels violence and mass displacement in Cameroon". United Nations. Retrieved 12 December 2021.
- "First wave". Science News. 28 February 2009. Archived from the original on 28 March 2012. Retrieved 23 September 2013.
- "Climate change, migration and critical international security considerations" (PDF). International Organization for Migration. Retrieved 13 October 2022.
- Rudolph, Lukas; Wagner, Markus (February 2022). "Europe's migration crisis: Local contact and out‐group hostility". European Journal of Political Research. 61 (1): 268–280. doi:10.1111/1475-6765.12455. hdl:20.500.11850/494987. ISSN 0304-4130. S2CID 234813782.
- Adger, W. Neil; Barnett, Jon; Brown, Katrina; Marshall, Nadine; O'Brien, Karen (February 2013). "Cultural dimensions of climate change impacts and adaptation". Nature Climate Change. 3 (2): 112–117. doi:10.1038/nclimate1666. ISSN 1758-6798.
- Environmental Migrants: Up To 1 Billion By 2050 Archived 19 December 2019 at the Wayback Machine, Centro Euro-Mediterraneo sui Cambiamenti Climactici (CMCC)
- The World Bank, "Part One: Chapter 2: Reducing Human Vulnerability: Helping People Help Themselves" (PDF), Managing social risks: Empower communities to protect themselves, archived (PDF) from the original on 7 May 2011, retrieved 29 August 2011, p. 109, WDR 2010 .
- Koubi, Vally (2019). "Climate Change and Conflict". Annual Review of Political Science. 22: 343–360. doi:10.1146/annurev-polisci-050317-070830.
- Burrows, Kate; Kinney, Patrick L. (April 2016). "Exploring the Climate Change, Migration and Conflict Nexus". International Journal of Environmental Research and Public Health. 13 (4): 443. doi:10.3390/ijerph13040443. PMC 4847105. PMID 27110806.
- Mach, Katharine J.; Kraan, Caroline M.; Adger, W. Neil; Buhaug, Halvard; Burke, Marshall; Fearon, James D.; Field, Christopher B.; Hendrix, Cullen S.; Maystadt, Jean-Francois; O'Loughlin, John; Roessler, Philip (2019). "Climate as a risk factor for armed conflict". Nature. 571 (7764): 193–197. Bibcode:2019Natur.571..193M. doi:10.1038/s41586-019-1300-6. ISSN 1476-4687. PMID 31189956. S2CID 186207310. Archived from the original on 11 August 2020. Retrieved 27 August 2020.
- Birkmann, Joern; Liwenga, Emma; Pandey, Rajiv; et al. "Chapter 8: Poverty, Livelihoods and Sustainable Developmen" (PDF). Climate Change 2022: Impacts, Adaptation and Vulnerability. Contribution of Working Group II to the Sixth Assessment Report of the Intergovernmental Panel on Climate Change. Intergovernmental Panel on Climate Change. Box 8.4.
- Spaner, J S; LeBali, H (October 2013). "The Next Security Frontier". Proceedings of the United States Naval Institute. 139 (10): 30–35. Archived from the original on 7 November 2018. Retrieved 23 November 2015.
- Perez, Ines (4 March 2013). "Climate Change and Rising Food Prices Heightened Arab Spring". Republished with permission by Scientific American. Environment & Energy Publishing, LLC. Archived from the original on 20 August 2018. Retrieved 21 August 2018.
- Rayner, S. and E.L. Malone (2001). "Climate Change, Poverty, and Intragernerational Equity: The National Leve". International Journal of Global Environmental Issues. 1. I (2): 175–202. doi:10.1504/IJGENVI.2001.000977.
- "Revised Estimates of the Impact of Climate Change on Extreme Poverty by 2030" (PDF). September 2020.
- Eastin, Joshua (1 July 2018). "Climate change and gender equality in developing states". World Development. 107: 289–305. doi:10.1016/j.worlddev.2018.02.021. ISSN 0305-750X. S2CID 89614518.
- Goli, Imaneh; Omidi Najafabadi, Maryam; Lashgarara, Farhad (9 March 2020). "Where are We Standing and Where Should We Be Going? Gender and Climate Change Adaptation Behavior". Journal of Agricultural and Environmental Ethics. 33 (2): 187–218. doi:10.1007/s10806-020-09822-3. ISSN 1573-322X. S2CID 216404045.
- McGregor, Deborah; Whitaker, Steven; Sritharan, Mahisha (1 April 2020). "Indigenous environmental justice and sustainability". Current Opinion in Environmental Sustainability. 43: 35–40. doi:10.1016/j.cosust.2020.01.007. ISSN 1877-3435.
- Ford, James D. (17 May 2012). "Indigenous Health and Climate Change". American Journal of Public Health. 102 (7): 1260–1266. doi:10.2105/AJPH.2012.300752. ISSN 0090-0036. PMC 3477984. PMID 22594718.
- Watts, Nick; Amann, Markus; Arnell, Nigel; Ayeb-Karlsson, Sonja; Belesova, Kristine; Boykoff, Maxwell; Byass, Peter; Cai, Wenjia; Campbell-Lendrum, Diarmid; Capstick, Stuart; Chambers, Jonathan (16 November 2019). "The 2019 report of The Lancet Countdown on health and climate change: ensuring that the health of a child born today is not defined by a changing climate". Lancet. 394 (10211): 1836–1878. doi:10.1016/S0140-6736(19)32596-6. ISSN 1474-547X. PMID 31733928. S2CID 207976337.
- Bartlett, Sheridan (2008). "Climate change and urban children: Impacts and implications for adaptation in low- and middle-income countries". Environment and Urbanization. 20 (2): 501–519. doi:10.1177/0956247808096125. S2CID 55860349.
- "WHO | The global burden of disease: 2004 update". WHO. Archived from the original on 24 March 2009.
- Lee, Jan (6 June 2013). "Understanding Environmental Justice Policies". Triple Pundit. Retrieved 12 November 2018.
- Hardy, Dean; Milligan, Richard; Heynen, Nik (December 2017). "Racial coastal formation: The environmental injustice of colorblind adaptation planning for sea-level rise". Geoforum. Amsterdam, Netherlands: Elsevier. 87: 62–72. doi:10.1016/j.geoforum.2017.10.005.
- I. Allison; et al. (2009). The Copenhagen Diagnosis, 2009: Updating the World on the Latest Climate Science (PDF).
- "Climate change: More than 3bn could live in extreme heat by 2070". BBC News. 5 May 2020. Archived from the original on 5 May 2020. Retrieved 6 May 2020.
- Xu, Chi; Kohler, Timothy A.; Lenton, Timothy M.; Svenning, Jens-Christian; Scheffer, Marten (26 May 2020). "Future of the human climate niche – Supplementary Materials". Proceedings of the National Academy of Sciences. 117 (21): 11350–11355. Bibcode:2020PNAS..11711350X. doi:10.1073/pnas.1910114117. ISSN 0027-8424. PMC 7260949. PMID 32366654.
- Pachauri, R.K.; Reisinger, A. (eds.). "3.3.3 Especially affected systems, sectors and regions". Contribution of Working Groups I, II and III to the Fourth Assessment Report of the Intergovernmental Panel on Climate Change. Geneva: IPCC. Archived from the original on 16 November 2018. Retrieved 19 February 2022.
- Mimura, N.; et al. (2007). "Chapter 16: Small Islands: Executive summary". In Parry, M.L.; et al. (eds.). Contribution of Working Group II to the Fourth Assessment Report of the Intergovernmental Panel on Climate Change. Cambridge, UK: IPCC; Cambridge University Press. ISBN 978-0521880107. Archived from the original on 14 October 2011. Retrieved 19 February 2022.
- "Climate change and the risk of statelessness" (PDF). May 2011. Retrieved 13 April 2012.
- Cities of the future: visualizing climate change to inspire action, current vs future cities, Crowther Lab, Department für Umweltsystemwissenschaften, Institut für integrative Biologie, ETH Zürich, zugegriffen: 11 July 2019.
- Understanding climate change from a global analysis of city analogues, Bastin J-F, Clark E, Elliott T, Hart S, van den Hoogen J, Hordijk I, et al. (2019), PLoS ONE 14(7): e0217592, Crowther Lab, Department for Environmental Systems Science, Institut for Integrative Biology, ETH Zürich, 10 July 2019.
- Tuholske, Cascade; Caylor, Kelly; Funk, Chris; Verdin, Andrew; Sweeney, Stuart; Grace, Kathryn; Peterson, Pete; Evans, Tom (12 October 2021). "Global urban population exposure to extreme heat". Proceedings of the National Academy of Sciences. 118 (41): e2024792118. Bibcode:2021PNAS..11824792T. doi:10.1073/pnas.2024792118. ISSN 0027-8424. PMC 8521713. PMID 34607944.
- "Synthesis report", Contribution of Working Groups I, II and III to the Fourth Assessment Report of the Intergovernmental Panel on Climate Change, Sec. 3.3.3 Especially affected systems, sectors and regions, archived from the original on 23 December 2018, retrieved 28 December 2018, in IPCC AR4 SYR 2007.
- Waha, Katharina (April 2017). "Climate change impacts in the Middle East and Northern Africa (MENA) region and their implications for vulnerable population groups". Regional Environmental Change. 17 (6): 1623–1638. doi:10.1007/s10113-017-1144-2. S2CID 134523218. Archived from the original on 23 July 2021. Retrieved 25 May 2020.
- Overland, Indra; Sagbakken, Haakon Fossum; Chan, Hoy-Yen; Merdekawati, Monika; Suryadi, Beni; Utama, Nuki Agya; Vakulchuk, Roman (December 2021). "The ASEAN climate and energy paradox". Energy and Climate Change. 2: 100019. doi:10.1016/j.egycc.2020.100019. hdl:11250/2734506.
- Schneider, S.H.; et al., "Ch 19: Assessing Key Vulnerabilities and the Risk from Climate Change", IPCC AR4 WG2 2007, Sec 19.3.3 Regional vulnerabilities, archived from the original on 23 December 2018, retrieved 28 December 2018
- BORENSTEIN, SETH; JORDANS, FRANK (4 August 2021). "This summer's disasters show climate change is very much 'a rich-country problem' now". Associated Press. Los Angeles Times. Archived from the original on 9 August 2021. Retrieved 9 August 2021.
- BORENSTEIN, SETH; JORDANS, FRANK (4 August 2021). "This year's summer of climate extremes hits wealthier places". Associaeted Press. Archived from the original on 9 August 2021. Retrieved 9 August 2021.
- Nicholls, Robert J.; et al. (2007). "Coastal systems and low-lying areas". In Parry, M.L.; et al. (eds.). Contribution of Working Group II to the Fourth Assessment Report of the Intergovernmental Panel on Climate Change. Cambridge, UK: IPCC; Cambridge University Press. pp. 315–356. ISBN 978-0521880107. Archived from the original on 22 November 2018. Retrieved 6 June 2018.
- Climate change: Sea level rise to affect 'three times more people' Archived 6 January 2020 at the Wayback Machine, BBC News, 30 October 2019
- Rising sea levels pose threat to homes of 300m people – study Archived 30 December 2019 at the Wayback Machine, The Guardian, 29 October 2019
- Kulp, Scott A.; Strauss, Benjamin H. (29 October 2019). "New elevation data triple estimates of global vulnerability to sea-level rise and coastal flooding". Nature Communications. 10 (1): 4844. Bibcode:2019NatCo..10.4844K. doi:10.1038/s41467-019-12808-z. ISSN 2041-1723. PMC 6820795. PMID 31664024. S2CID 204962583.
- IPCC (2007). "3.3.1 Impacts on systems and sectors. In (section): Synthesis Report. In: Climate Change 2007: Synthesis Report. Contribution of Working Groups I, II and III to the Fourth Assessment Report of the Intergovernmental Panel on Climate Change (Core Writing Team, Pachauri, R.K and Reisinger, A. (eds.))". Book version: IPCC, Geneva, Switzerland. This version: IPCC website. Archived from the original on 3 November 2018. Retrieved 10 April 2010.
- "'A Climate Emergency Unfolding Before Our Eyes.' Arctic Sea Ice Has Shrunk to Almost Historic Levels". Time. Archived from the original on 9 October 2020. Retrieved 11 October 2020.
- Rasheed Hassan, Hussain; Cliff, Valerie (24 September 2019). "For small island nations, climate change is not a threat. It's already here". World Economic Fourm. Retrieved 28 January 2021.
- Barnett, Jon; Adger, W. Neil (2003). "Climate Dangers and Atoll Countries". Climatic Change. 61 (3): 321–337. doi:10.1023/B:CLIM.0000004559.08755.88. ISSN 0165-0009. S2CID 55644531.
- Church, John A.; White, Neil J.; Hunter, John R. (2006). "Sea-level rise at tropical Pacific and Indian Ocean islands". Global and Planetary Change. 53 (3): 155–168. Bibcode:2006GPC....53..155C. doi:10.1016/j.gloplacha.2006.04.001.
- Mimura, N (1999). "Vulnerability of island countries in the South Pacific to sea level rise and climate change". Climate Research. 12: 137–143. Bibcode:1999ClRes..12..137M. doi:10.3354/cr012137.
- Tsosie, Rebecca (2007). "Indigenous People and Environmental Justice:The Impact of Climate Change". University of Colorado Law Review. 78: 1625.
- Mortreux, Colette; Barnett, Jon (2009). "Climate change, migration and adaptation in Funafuti, Tuvalu". Global Environmental Change. 19 (1): 105–112. doi:10.1016/j.gloenvcha.2008.09.006.
- Pester, Patrick (30 August 2021). "Could climate change make humans go extinct?". Live Science. Archived from the original on 30 August 2021. Retrieved 31 August 2021.
- "Human Civilization Will Crumble by 2050 If We Don't Stop Climate Change Now, New Paper Claims". livescience.com. 4 June 2019. Retrieved 13 October 2022.
- Steffen, Will; Persson, Åsa; Deutsch, Lisa; Zalasiewicz, Jan; Williams, Mark; Richardson, Katherine; Crumley, Carole; Crutzen, Paul; Folke, Carl; Gordon, Line; Molina, Mario; Ramanathan, Veerabhadran; Rockström, Johan; Scheffer, Marten; Schellnhuber, Hans Joachim; Svedin, Uno (12 October 2011). "The Anthropocene: From Global Change to Planetary Stewardship". AMBIO. 40 (7): 739–761. doi:10.1007/s13280-011-0185-x. ISSN 1654-7209. PMC 3357752. PMID 22338713.
- Elander, Ingemar; Granberg, Mikael; Montin, Stig (1 October 2022). "Governance and planning in a 'perfect storm': Securitising climate change, migration and Covid-19 in Sweden". Progress in Planning. 164: 100634. doi:10.1016/j.progress.2021.100634. ISSN 0305-9006. PMC 9535694. PMID 36217494.
- Dar, William D.; Laxmipathi Gowda, C. L. (1 March 2013). "Declining Agricultural Productivity and Global Food Security" (PDF). Journal of Crop Improvement. 27 (2): 242–254. doi:10.1080/15427528.2011.653097. ISSN 1542-7528. S2CID 84235247.
- Katona, Peter; Sullivan, John P.; Intriligator, Michael D. (21 January 2010). Global Biosecurity: Threats and Responses. Routledge. ISBN 978-1-135-27302-6.
- "Climate endgame: risk of human extinction 'dangerously underexplored'". The Guardian. 1 August 2022. Retrieved 15 September 2022.
- Howell, Rachel; Allen, Simon (1 April 2017). "People and Planet: Values, Motivations and Formative Influences of Individuals Acting to Mitigate Climate Change". Environmental Values. 26 (2): 131–155. doi:10.3197/096327117X14847335385436. hdl:20.500.11820/1e519e9b-658b-4d9d-99f5-0c446494d35a.
- Howell, Rachel A. (1 February 2013). "It's not (just) "the environment, stupid!" Values, motivations, and routes to engagement of people adopting lower-carbon lifestyles". Global Environmental Change. 23 (1): 281–290. doi:10.1016/j.gloenvcha.2012.10.015. hdl:20.500.11820/fd61119b-7eb4-414e-9aa3-c524189f1eda. ISSN 0959-3780.
- "Wieder weltweite Proteste für das Klima". n-tv (in German). Retrieved 11 June 2022.
- "Klimaforscher Schellnhuber: "Enorme Sorgen" wegen Kohlekommission". heise online (in German). Retrieved 11 June 2022.
- "Economy and Finance for a Just Future on a Thriving Planet". Economy and Finance for a Just Future on a Thriving Planet. 29 May 2022. Retrieved 13 October 2022.
Sources
- IPCC AR4 WG1 (2007), Solomon, S.; Qin, D.; Manning, M.; Chen, Z.; Marquis, M.; Averyt, K.B.; Tignor, M.; Miller, H.L. (eds.), Climate Change 2007: The Physical Science Basis, Contribution of Working Group I to the Fourth Assessment Report of the Intergovernmental Panel on Climate Change, Cambridge University Press, ISBN 978-0-521-88009-1 (pb: 978-0-521-70596-7).
- IPCC AR4 WG2 (2007), Parry, M.L.; Canziani, O.F.; Palutikof, J.P.; van der Linden, P.J.; Hanson, C.E. (eds.), Climate Change 2007: Impacts, Adaptation and Vulnerability, Contribution of Working Group II to the Fourth Assessment Report of the Intergovernmental Panel on Climate Change, Cambridge University Press, ISBN 978-0-521-88010-7 (pb: 978-0-521-70597-4).
- IPCC AR4 SYR (2007), Core Writing Team; Pachauri, R.K; Reisinger, A. (eds.), Climate Change 2007: Synthesis Report, Contribution of Working Groups I, II and III to the Fourth Assessment Report of the Intergovernmental Panel on Climate Change, Geneva, Switzerland: IPCC, ISBN 978-92-9169-122-7.
- IPCC SREX (2012), Field, C.B.; et al. (eds.), Managing the Risks of Extreme Events and Disasters to Advance Climate Change Adaptation (SREX), Cambridge University Press, archived from the original on 19 December 2012. Summary for Policymakers available in Arabic, Chinese, French, Russian, and Spanish.
- IPCC AR5 WG1 (2013), Stocker, T.F.; et al. (eds.), Climate Change 2013: The Physical Science Basis. Working Group 1 (WG1) Contribution to the Intergovernmental Panel on Climate Change (IPCC) 5th Assessment Report (AR5), Cambridge University Press. Climate Change 2013 Working Group 1 website.
- IPCC AR5 WG2 A (2014), Field, C.B.; et al. (eds.), Climate Change 2014: Impacts, Adaptation, and Vulnerability. Part A: Global and Sectoral Aspects. Contribution of Working Group II (WG2) to the Fifth Assessment Report (AR5) of the Intergovernmental Panel on Climate Change (IPCC), Cambridge University Press, archived from the original on 16 April 2014. Archived
- IPCC (2018). Masson-Delmotte, V.; Zhai, P.; Pörtner, H. O.; Roberts, D.; et al. (eds.). Global Warming of 1.5°C. An IPCC Special Report on the impacts of global warming of 1.5°C above pre-industrial levels and related global greenhouse gas emission pathways, in the context of strengthening the global response to the threat of climate change, sustainable development, and efforts to eradicate poverty (PDF). Intergovernmental Panel on Climate Change.
- IPCC (2019). Pörtner, H.-O.; Roberts, D.C.; Masson-Delmotte, V.; Zhai, P.; et al. (eds.). IPCC Special Report on the Ocean and Cryosphere in a Changing Climate (PDF). IPCC.
- IPCC (2019). Shukla, P.R.; Skea, J.; Calvo Biendia, E.; Masson-Delmotte, V.; et al. (eds.). Climate Change and Land. An IPCC Special Report on Climate Change, Desertification, Land Degradation, Sustainable Land Management, Food Security, and Greenhouse gas fluxes in Terrestrial Ecosystems (PDF). In press.
- IPCC (2019). "Summary for Policymakers" (PDF). IPCC SRCCL 2019. pp. 3–34.
This article incorporates public domain material from Kennedy, C.H. (10 July 2012). ClimateWatch Magazine » State of the Climate in 2011: Highlights. NOAA. Archived from the original on 10 May 2013. Retrieved 20 March 2013.
This article incorporates public domain material from Glossary of Climate Change Terms: Climate Change: US EPA. US Environmental Protection Agency (EPA) Climate Change Division. 14 June 2012.
External links

- IPCC Working Group I (WG I). Intergovernmental Panel on Climate Change group which assesses the physical scientific aspects of the climate system and climate change.
- Climate from the World Meteorological Organization
- Climate change UN Department of Economic and Social Affairs Sustainable Development
- Effects of climate change from the Met Office
- United Nations Environment Programme and climate change