Coinfection
Coinfection | |
---|---|
![]() | |
Coinfection Hepatitis B virus and Hepatitis D virus -Gel photograph of different HDV product, Lane 1: HDV negative; Lane 2: Ladder marker; Lanes 3-5: showing HDV positive samples (137-base pairs); Lane 6 showing negative control; Lane 7 is positive control | |
![]() | |
Pronunciation |
|
Specialty | Infectious disease |
Coinfection is the simultaneous infection of a host by multiple pathogen species. In virology, coinfection includes simultaneous infection of a single cell by two or more virus particles. An example is the coinfection of liver cells with hepatitis B virus and hepatitis D virus, which can arise incrementally by initial infection followed by superinfection.Global prevalence or incidence of coinfection among humans is unknown, but it is thought to be commonplace,[1] sometimes more common than single infection.[2] Coinfection with helminths affects around 800 million people worldwide.[3]
Coinfection is of particular human health importance because pathogen species can interact within the host. The net effect of coinfection on human health is thought to be negative.[4] Interactions can have either positive or negative effects on other parasites. Under positive parasite interactions, disease transmission and progression are enhanced and this is also known as syndemism. Negative parasite interactions include microbial interference when one bacterial species suppresses the virulence or colonisation of other bacteria, such as Pseudomonas aeruginosa suppressing pathogenic Staphylococcus aureus colony formation.[5] The general patterns of ecological interactions between parasite species are unknown, even among common coinfections such as those between sexually transmitted infections.[6] However, network analysis of a food web of coinfection in humans suggests that there is greater potential for interactions via shared food sources than via the immune system.[7]
A globally common coinfection involves tuberculosis and HIV. In some countries, up to 80% of tuberculosis patients are also HIV-positive.[8] The potential for dynamics of these two infectious diseases to be linked has been known for decades.[9] Other common examples of coinfections are AIDS, which involves coinfection of end-stage HIV with opportunistic parasites[10] and polymicrobial infections like Lyme disease with other diseases.[11] Coinfections sometimes can epitomize a zero sum game of bodily resources, and precise viral quantitation demonstrates children co-infected with rhinovirus and respiratory syncytial virus, metapneumovirus or parainfluenza virus have lower nasal viral loads than those with rhinovirus alone.[12]
Poliovirus
Coinfections appear to be common and several pathways have been identified for transmitting multiple virions to a single host cell.[13] These include transmission by virion aggregates, transmission of viral genomes within membrane vesicles, and transmission by bacteria bound by several viral particles. Drake demonstrated that poliovirus is able to undergo multiplicity reactivation.[14] That is, when polioviruses were irradiated with UV light and allowed to undergo multiple infections of host cells, viable progeny could be formed even at UV doses that inactivated the virus in single infections. Poliovirus can undergo genetic recombination when at least two viral genomes are present in the same host cell. Kirkegaard and Baltimore[15] presented evidence that RNA-dependent RNA polymerase (RdRP) catalyzes recombination by a copy choice mechanism in which the RdRP switches between (+)ssRNA templates during negative strand synthesis. Recombination in RNA viruses appears to be an adaptive mechanism for transmitting an undamaged genome to virus progeny.[16][17]
Other coinfections
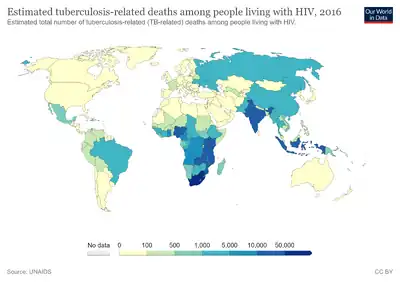
- Anaplasmosis
- Bacteriophage coinfection
- GB virus C
- HIV-HCV coinfection
- HIV-TB coinfection (enhances TB transmission and lethality)
- Hepatitis D
- Hookworm-malaria coinfection
- Mansonella perstans
- Trichuriasis
- Chikungunya and Dengue coinfection
- Dengue and HIV coinfection (suppresses HIV)
- Chagas and HIV coinfection
- Most sexually transmitted diseases and HIV (enhance HIV transmission)
- Some COVID-19 patients, or those who were ill with other coronaviruses, can be co-infected with seasonal influenza (flu) viral strains, certain viral strains that cause the common cold, or can be co-infected with bronchitis or pneumonia from another bacterial or viral micro-organism. Even more dangerous, some of them could already have conditions like tuberculosis or active AIDS that make patients very vulnerable.
See also
References
- ↑ Cox, FE (2001). "Concomitant infections, parasites and immune responses" (PDF). Parasitology. 122. Suppl: S23–38. doi:10.1017/s003118200001698x. PMID 11442193. S2CID 150432. Archived (PDF) from the original on 2019-10-03. Retrieved 2022-08-14.
- ↑ Petney, TN; Andrews, RH (1998). "Multiparasite communities in animals and humans: frequency, structure and pathogenic significance". International Journal for Parasitology. 28 (3): 377–93. doi:10.1016/S0020-7519(97)00189-6. PMID 9559357.
- ↑ Crompton, DW (1999). "How much human helminthiasis is there in the world?". The Journal of Parasitology. 85 (3): 397–403. doi:10.2307/3285768. JSTOR 3285768. PMID 10386428.
- ↑ Griffiths, EC; Pedersen, ABP; Fenton, A; Petchey, OP (2011). "The nature and consequences of coinfection in humans". Journal of Infection. 63 (3): 200–206. doi:10.1016/j.jinf.2011.06.005. PMC 3430964. PMID 21704071.
- ↑ Hoffman, L. R.; Deziel, E.; D'argenio, D. A.; Lepine, F.; Emerson, J.; McNamara, S.; Gibson, R. L.; Ramsey, B. W.; Miller, S. I. (2006). "Selection for Staphylococcus aureus small-colony variants due to growth in the presence of Pseudomonas aeruginosa". Proceedings of the National Academy of Sciences. 103 (52): 19890–5. Bibcode:2006PNAS..10319890H. doi:10.1073/pnas.0606756104. PMC 1750898. PMID 17172450.
- ↑ Shrestha, S. (2011). "Influence of host genetic and ecological factors in complex concomitant infections – relevance to sexually transmitted infections". Journal of Reproductive Immunology. 92 (1–2): 27–32. doi:10.1016/j.jri.2011.09.001. PMID 22019002.
- ↑ Griffiths, E.; Pedersen, A.; Fenton, A.; Petchey, O. (2014). "Analysis of a summary network of co-infection in humans reveals that parasites interact most via shared resources". Proceedings of the Royal Society B. 281 (1782): 20132286. doi:10.1098/rspb.2013.2286. PMC 3973251. PMID 24619434.
- ↑ "Tuberculosis and HIV". World Health Organization. Archived from the original on July 21, 2006.
- ↑ Di Perri, G; Cruciani, M; Danzi, MC; Luzzati, R; De Checchi, G; Malena, M; Pizzighella, S; Mazzi, R; et al. (1989). "Nosocomial epidemic of active tuberculosis among HIV-infected patients". Lancet. 2 (8678–8679): 1502–4. doi:10.1016/s0140-6736(89)92942-5. PMID 2574778. S2CID 5608415.
- ↑ Lawn, SD (2004). "AIDS in Africa: the impact of coinfections on the pathogenesis of HIV-1 infection". Journal of Infection. 48 (1): 1–12. doi:10.1016/j.jinf.2003.09.001. PMID 14667787.
- ↑ Mitchell, PD; Reed, KD; Hofkes, JM (1996). "Immunoserologic evidence of coinfection with Borrelia burgdorferi, Babesia microti, and human granulocytic Ehrlichia species in residents of Wisconsin and Minnesota". Journal of Clinical Microbiology. 34 (3): 724–7. doi:10.1128/JCM.34.3.724-727.1996. PMC 228878. PMID 8904446.
- ↑ Waghmare, A; Strelitz, B; Lacombe, K; Perchetti, GA; Nalla, A; Rha, B; Midgley, C; Lively, JY; Klein, EJ; Kuypers, J; Englund, JA (2019). "Rhinovirus in Children Presenting to the Emergency Department: Role of Viral Load in Disease Severity and Co-Infections". Open Forum Infectious Diseases. 6 (10): S915–S916. doi:10.1093/ofid/ofz360.2304. PMC 6810026.
- ↑ Aguilera ER, Pfeiffer JK. Strength in numbers: Mechanisms of viral co-infection. Virus Res. 2019;265:43-46. doi:10.1016/j.virusres.2019.03.003
- ↑ Drake JW (August 1958). "Interference and multiplicity reactivation in polioviruses". Virology. 6 (1): 244–64. doi:10.1016/0042-6822(58)90073-4. PMID 13581529.
- ↑ Kirkegaard K, Baltimore D (November 1986). "The mechanism of RNA recombination in poliovirus". Cell. 47 (3): 433–43. doi:10.1016/0092-8674(86)90600-8. PMC 7133339. PMID 3021340.
- ↑ Barr JN, Fearns R (June 2010). "How RNA viruses maintain their genome integrity". The Journal of General Virology. 91 (Pt 6): 1373–87. doi:10.1099/vir.0.020818-0. PMID 20335491.
- ↑ Bernstein H, Bernstein C, Michod RE (January 2018). "Sex in microbial pathogens". Infection, Genetics and Evolution. 57: 8–25. doi:10.1016/j.meegid.2017.10.024. PMID 29111273.
External links
Classification |
|
---|