Axial twist theory
The axial twist hypothesis is a theory put forward to explain a range of unusual aspects of the body plan of vertebrates (including humans).[1] It proposes that the rostral part of the head is "turned around" regarding the rest of the body.[2] This end-part consists of the face (eyes, nose, and mouth) as well as part of the brain (cerebrum and thalamus). According to the theory, the vertebrate body has a left-handed chirality.
Axial twist | |
---|---|
![]() Schema of the development of the axial twist. Developmental phases are (from top to bottom): (1) the embryo turns on its left side; (2) the anterior head grows in the same direction, but the rest of the body grows oppositely into a twist. So that ultimately (3) external bilateral symmetry is regained. Note, that there is no evolutionary pressure for internal symmetry so the heart (and other organs) remain asymmetric. | |
Details | |
System | vertebrate body plan |
Anatomical terminology |
The theory gives a phenomenal explanation and addresses how and when the twist between the end of the head and the rest of the body develops. It addresses the possible evolutionary history. The goal is to make testable predictions. For example, the theory predicted the aurofacial asymmetry, which was then found empirically.[3]
Explained phenomena include:
- Contralateral organization of the brain
- Left-sided orientation of the heart
- Asymmetric position of the gastrointestinal tract, the liver, and the pancreas
- Optic chiasm
- chiasm of the trochlear nerve
- Non-crossed olfactory tract
- Aurofacial asymmetry
- Yakovlevian torque
- Asymmetry of the thoracal vertebra
According to the axial twist developmental model, the anterior part of the head turns against the rest of the body, except for the inner organs. Due to this twist, the forebrain and face are turned around such that left and right, but also anterior and posterior are flipped in the adult vertebrate. There are some popular science videos and podcasts on the topic.[4][5]
History
The idea of a twist responded to severe inconsistencies in the prevailing scientific hypotheses to explain the contralateral organization of the forebrain (cerebrum and thalamus). Briefly, the visual map theory by Santiago Ramón y Cajal proposes that the optic chiasm restores the retinal image on the visual cortex,[6][7] but the loop of the optic radiation destroys this potential repair again, so there is no such consistency.[8] The parcellation theory proposes that an increasing brain size can conserve coincidental contralateral organization,[9] but does not explain the optic chiasm, nor that the pattern is conserved across all extant vertebrates regardless of brain size. Functional[10][11] or topological[12] explanations fail because same-side connections are just as important as crossing ones.[13] Moreover, these explanations leave open why the brain stem, including the cerebrum, has a same-side organization.
The idea of a twist was proposed two times independently. It does not suffer from these problems. The first presentation occurred at a conference in the 1970s by Marcel Kinsbourne,[14] but remained widely unknown. The first scientific publication in the form of a falsifiable scientific theory was made in 2012 by Marc de Lussanet and Jan Osse.[1] Kinsbourne subsequently also published his ideas in 2013.[2] In 2019, a novel kind of facial asymmetry, the aurofacial asymmetry, was predicted and confirmed on the basis of the axial twist theory.[3]
Kinsbourne developed his ideas from the dorsoventral inversion hypothesis by the early 19th-century naturalist Étienne Geoffroy Saint-Hilaire, proposing that the anterior head was the part of the head that was not inverted. He also stressed that the axial twist concerns all chordates. This proposal has the problem that such a turn does not occur during embryological development and has no evolutionary foundation.
De Lussanet and Osse used comparative functional morphology and embryonic development as a starting point to arrive at the axial twist hypothesis.
Embryology
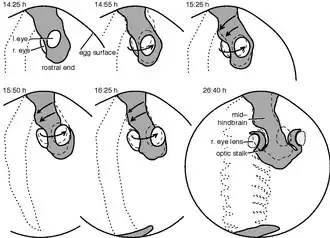
Although the embryological development of the axial twist has not been studied explicitly, there are clear indications from the zebrafish and the chick.[1] The twist begins briefly after the neurulation and commences in a rostrocaudal (front-to-tail) direction.
Philipp Keller's group traced each cell of developing zebrafish embryos until the first heartbeat.[15] Tracing the movements of the cells in the future eye region and the hind part of the head, revealed opposite movement directions, in accordance with an axial twist. Whereas the left-eye eye-region cells tendentially moved outwards and downwards (ventrally), those of the right eye region moved out- and upwards, as visualized by a time-lapse video.[16] On the other hand, the surface cells of the hind side of the head moved to the left, consistent with an axial twist.[1]
The chick development has been studied well. The development is usually described according to the Hamburger–Hamilton stages.[17] The twisting begins during stage 6 on the rostral side of the head region[18] and commences until stage 14 towards the heart region.[19] Whereas the anterior head region rotates with the right side moving in an upward direction and the left side downward, the heart region moves in the opposite direction. In the end, the chick is turned on its right side, whereas the heart, not taking part in the twisting, has landed on the left side of the body.[1]
Growth after birth
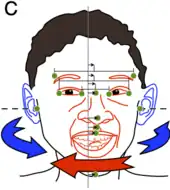
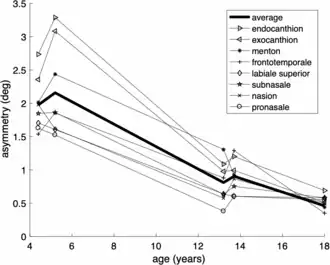
In humans, this process of asymmetric growth that leads to approximate bilateral symmetry continues until puberty.[3] This was shown from an analysis of the so-called aurofacial asymmetry. The aurofacial asymmetry expresses the position of the face (eyes, nose, mouth) with respect to the plane perpendicular to the axis through the ears. As shown in the graph, the asymmetry decreases until the age of 13. Since the axial twist is located between the ears and the face, it is predicted that the face grows from the left to the midline, as is indeed the case.
Genetic mechanisms
The axial twist emerges through opposite asymmetric development. This can be observed as a wave moving across the embryo from anterior to posterior. It is now well established, that the Nodal signaling cascade and the right-to-left flow produced by ciliated cells in the primitive streak are central in setting up the asymmetric organization. Three aspects of this growth wave are:[1]
- the asymmetric growth (left-side-turn) in the anterior tip of the primitive node (aka Spemann-Mangold organizer in amphibians or Hensen's node in birds),[18][20]
- the left-right situs of the organs and
- the right-side-turn of the body.
Nodal, FGF8, and shh as well as the motor protein Kif5c have been associated with the asymmetric growth of the anterior primitive node, although only Nodal seems to be expressed before the initiation of the asymmetry[20][21]
The Nodal and cSnR genes control the asymmetric development of the heart.[22]
The right-side-turn of the body is tightly linked to the same genetic mechanisms.[22]
Developmental malformations
In holoprosencephaly, the hemispheres of the cerebrum (or part of it) are not aligned on the left and right sides but only on the frontal and occipital sides of the skull, and the head usually remains very small. According to the axial twist theory, this represents an extreme case of Yakovlevian torque,[23] and may occur when the cerebrum does not turn during early embryology.
Cephalopagus or janiceps twins are conjoined twins who are born with two faces, one on either side of the head. These twins have two brains and two spinal cords, but these are located on the left and the right side of the body.[24] According to the axial twist model, the two nervous systems could not turn due to the complex configuration of the body and therefore remained on either side.[1]
Evolution
The axial twist is thought to have evolved in a common ancestor of all vertebrates. The reason for that is that even the most distant clades of vertebrates — the agnathan lamprey and hagfish — possess an optic chiasm and contralateral brain organization,[25] as well as a left-sided heart and asymmetric bowels.[26] Also, every vertebrate has a contralateral organization of the forebrain.[25] Fossil skull impressions of early vertebrates from the Ordovician and later show the presence of an optic chiasm.[27]
Twisting and asymmetric development are well known from other deuterostomes — such as Hemichordata, Echinodermata, Cephalochordata, and Tunicata. Turning toward the side or upside-down also occurs frequently in these clades (e.g. sea stars which turn their mouth downwards after the larva has briefly settled with the mouth turned up, or the adult lancelet which buries obliquely with its mouth turned up, or many fish which tend to turn around when feeding from the water surface).
Thus, the axial twist evolved in an ancestor to all vertebrates, and possibly of all chordates or even in an early deuterostome.
Morphology
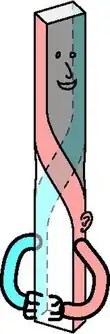
The axial twist takes place in the early embryo of a vertebrate. There is an evolutionary pressure for animals towards bilateral symmetry, due to sexual selection (better looks to potential mates) and functional selection (e.g., better locomotion). The evolutionary pressure decreases with better symmetry. The pressure also decreases as a body part is less associated with the body surface and the locomotor system.
Consequently, the axial twist theory predicts that small, systematic asymmetries remain on the outside of the body and that these asymmetries are larger on the inside of the body.
Contralateral organization of the brain, Yakovlevian torque, and spinal asymmetry
The forebrain (cerebrum and thalamus) predominantly represents the opposite side of the body (and the visual world). However, many functions require information from both sides of the body, and so the contralateral representation is by no means absolute. Rather, almost every region of the brain connects to both sides of the body.
The Yakovlevian torque[28] (aka "counterclockwise brain torque"[30] refers to an anatomical peculiarity of the normal brain. On average, the frontal lobes are asymmetric to the left (the right lobe appears slightly larger than the left), whereas the occipital lobe is asymmetric to the right; the central sulcus and temporal lobe of the right cortical hemisphere are further to the front than those on the left. Overall, these asymmetries are equivalent to a slight rotation of the cerebrum (i.e. torque). Such a rotation is exactly as predicted by the axial twist theory, given that the cerebrum is not a superficial structure.
The torque is also known as "occipital bending"[31] if it is more strongly expressed on the occipital side than on the frontal side.
The spine is slightly asymmetric. In healthy subjects, the thoracic vertebrae (vertebra T6-T12) were on average asymmetric, such that the mid-line points to the right (2.5° in T6).[29] Thus, the Yakovlevian torque and the spinal asymmetry are in opposite direction, just as predicted by the axial twist theory.
Decussations of the central nervous system
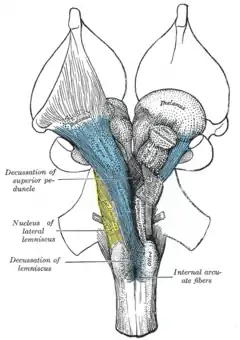

If the right forebrain represents predominantly the left body and the left forebrain the right body, there should be positions of major nerve crossings behind the forebrain.
Anatomically, the contralateral organization of the forebrain is manifested by major decussations (based upon the Latin notation for ten, 'deca,' as an uppercase 'X') and chiasmas (after the Greek uppercase letter 'Χ,' chi). A decussation denotes a crossing of bundles of axonal fibers inside the central nervous system. As a result of such decussations: The efferent connections of the cerebrum to the basal ganglia, the cerebellum, and the spinal cord are crossed; and the afferent connections from the spine, the cerebellum, and the pons to the thalamus are crossed.[25] Thus, motor, somatosensory, auditory, and visual primary regions in the forebrain predominantly represent the contralateral side of the body.
Most afferent and efferent connections of the forebrain have bilateral components, especially outside the primary sensory and motor regions. As a result, hemiplegia that is acquired in early childhood can sometimes be completely compensated over time.
The visual sensory and motor system
Four of the cranial nerves serve the eye directly: one sensory and three motor nerves. The optic nerve is sensory and crosses the midline in the optic chiasm. The oculomotor nerve, trochlear nerve, and abducens nerve are motor nerves that control one or more of the eye muscles. The oculomotor nerve crosses the midline before leaving the central nervous system. The trochlear nerve crosses the midline in a chiasma on the dorsal side and the abducens innervates an eye muscle on the same side.[32]: Fig. 17.8
In the light of the axial twist theory, this complicated pattern can be understood. The eyes, like the mouth and the nose originate from the anterior head region, i.e. in front of the twist. The only cranial nerve that originates from the forebrain is the olfactory nerve. All other cranial nerves originate from regions of the central nervous system that lie behind the twist.[1]
The optic nerve inserts on the optic tectum of the midbrain. In tetrapods and bony fish it also branches off to the LGN of the thalamus in the forebrain, but not in other vertebrates such as sharks and skates). In sharks, the visual center in the cerebrum obtains its fibers from the optic tectum. on the way these fibers cross the midline again so that each hemisphere of the cerebrum of sharks represents the eye on the same side.[33][34] Thus, the optic tract largely follows the prediction of the axial twist theory. The branch towards the LGN has been acquired later in the evolution and thus makes an exception.
The oculomotor and trochlear nerves originate from the midbrain and cross the midline as predicted by the theory.
The abducens nucleus is located in the pons. The abducens nerve innervates the lateral rectus muscle of the eye in most vertebrates, except lampreys and hagfishes. It thus seems that the lateral rectus muscle evolved later and independently of the other eye muscles, and presents an exception to the axial twist model.
The olfactory system
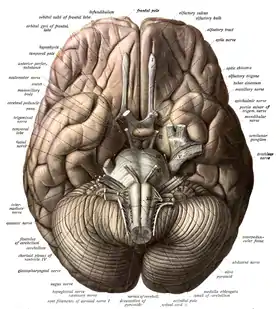
The olfactory tracts run parallel to the optic tract but do not form a chiasm. Accordingly, each olfactory bulb connects to the same-side centers of the frontal cerebrum. This is entirely consistent with the axial twist theory because the nose is part of the anterior head region which twists along with the forebrain. Since the primary olfactory centers are at home in the cerebrum (olfaction is the only sense that originates in the cerebrum), each olfactory lobe is predicted to be represented by the cerebrum on the same side.
Aurofacial asymmetry
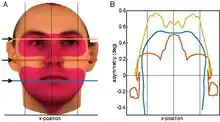
The axial twist theory predicts that even in adults, a small aurofacial asymmetry remains.[3] Since the ears are behind the twist and the face is in front of the twist, the ears can be used as a reference. Three-dimensional facial scans have been computed for 200 healthy adults.[35][36] For this sample, the asymmetry of the face with respect to the ears was on average about 0.5°, meaning that the right side of the face is on average slightly larger than the left side. This aurofacial asymmetry is much larger (4°) in small children.[3]
Left heart and stomach, right liver, asymmetric bowels

The inner organs of the trunk are the regions on the body that are least mechanically associated with locomotion and the external body, and so are predicted by the axial twist theory to be the most asymmetric regions of the body. Other bilaterally symmetric animals such as insects and annelids are bilaterally symmetric also on the inside. The asymmetric development of the heart is well-researched.[22][37]
The question of why the heart should have a left-sided orientation is frequently asked.[38] Various explanations exist, but it seems that the axial twist is presently the only scientific theory that addresses the question.
The asymmetric orientation and position of the gastrointestinal tract, the liver, and the pancreas have been studied much less than the heart, probably because the heart is very prominent already in the early embryonic stages and everyone is aware of their heart throughout life.
Relation to other theories
There are no other theories or hypotheses that explain the entire spectrum covered by the axial twist theory. Several hypotheses have been proposed to explain (certain aspects of) the contralateral brain (i.e. the left forebrain representing mostly the right body and the right forebrain representing mostly the left body).
No hypotheses have been published for the asymmetry of the spine. There is no other theory to explain the left position of the heart or the asymmetric orientation of the bowels and related organs.
The Yakovlevian torque is often thought to reflect the lateralization of specific functions in the brain.[28]
Inversion hypothesis
In 1822 the French zoologist Étienne Geoffroy Saint-Hilaire noted that the organization of dorsal and ventral structures in the crayfish (an arthropod) is opposite that of mammals, and he proposed that mammals and other vertebrates are turned upside down.[39][40] As explained above, Marcel Kinsbourne proposed that the body (soma) but not the anterior head is inverted (hence somatic twist hypothesis).[2]
There is molecular evidence for the inversion hypothesis in almost all groups of deuterostomes.[41][42]
Open questions
The axial twist theory is a novel scientific discipline and very few scientific papers have presently addressed it directly.[1][2][8][3] Although a considerable volume of research exists on the genetic and embryological mechanisms of asymmetric development, an open question is how the twist is initiated and how the inversion of the left-right and up-down axes in the anterior head region is established.
The embryology of the twisting has been addressed only rudimentary in the chick and the zebrafish.[1] The differences in timing and mechanisms across the vertebrate clades are completely unknown.
The evolution of the axial twist is an open question. The founders of de axial twist idea (de Lussanet & Osse, and Kinsbourne) agree that the axial twist is universal in vertebrates and probably is a feature of all chordates.[1][2] Although the asymmetric development of other chordates such as the Lancelet has been studied in detail, no study has analysed this development in the light of the axial twist theory. Moreover, even other deuterostomes, i.e. the echinoderms (sea stars, sea lilies, etc.) show a marked asymmetric development and even an axial twist[43] This twist has remarkable similarities to that in vertebrates, but no study has addressed this at present. Lastly, the asymmetric and twisted development is well known from gastropods and the relation to asymmetric development in vertebrates is an important question.
It has been proposed that problems in the axial twist development may play a central role in developmental malformations such as holoprosencephaly[1] and skoliosis[3] but these have not been looked into.
References
- de Lussanet, M. H. E.; Osse, J. W. M. (2012). "An ancestral axial twist explains the contralateral forebrain and the optic chiasm in vertebrates". Animal Biology. 62 (2): 193–216. arXiv:1003.1872. doi:10.1163/157075611X617102. S2CID 7399128.
- Kinsbourne, M. (2013). "Somatic twist: a model for the evolution of decussation". Neuropsychology. 27 (5): 511–515. doi:10.1037/a0033662. PMID 24040928. S2CID 11646580.
- de Lussanet, M. H. E. (2019). "Opposite asymmetries of face and trunk and of kissing and hugging, as predicted by the axial twist hypothesis". PeerJ. 7: e7096. doi:10.7717/peerj.7096. PMC 6557252. PMID 31211022.
- "Your head might be on sideways". YouTube. 2020. Retrieved 2020-02-09.
- Chris Smith (11 Aug 2023). "Question of the Week". www.thenakedscientists.com (Podcast). Cambridge University. Event occurs at 23:30. Retrieved 14 Aug 2023.
- Ramón y Cajal, Santiago (1898). "Estructura del quiasma óptico y teoría general de los entrecruzamientos de las vías nerviosas. (Structure of the Chiasma opticum and general theory of the crossing of nerve tracks)" [Die Structur des Chiasma opticum nebst einer allgemeine Theorie der Kreuzung der Nervenbahnen (German, 1899, Verlag Joh. A. Barth)]. Rev. Trim. Micrográfica (in Spanish). 3: 15–65.
- Llinás, R. R. (2003). "The contribution of Santiago Ramón y Cajal to functional neuroscience". Nat. Rev. Neurosci. 4 (1): 77–80. doi:10.1038/nrn1011. PMID 12511864. S2CID 30442863.
- de Lussanet, M. H. E.; Osse, J. W. M. (2015). "Decussation as an axial twist: A comment on Kinsbourne (2013)" (PDF). Neuropsychology. 29 (5): 713–714. doi:10.1037/neu0000163. PMID 25528610. Archived from the original (PDF) on 2021-07-14. Retrieved 2020-01-06.
- Ebbesson, Sven O. E.; Ito, Hironobu (1980). "Bilateral retinal projections in the black piranah (Serrasalmus niger)". Cell Tissue Res. 213 (3): 483–495. doi:10.1007/BF00237893. PMID 7448850. S2CID 2406618.
- Bertin, René J. V. (1994). Natural smartness in hypothetical animals: Of paddlers and glow balls (PDF) (PhD thesis). Utrecht: Utrecht University.
- Loeb, Jacques (1918). Forced movements, tropisms and animal conduct. Philadelphia and London: Lippincott. p. 209.
- Shinbrot, T.; Young, W. (2008). "Why decussate? Topological constraints on 3D wiring". Anatomical Record. 291 (10): 1278–1292. doi:10.1002/ar.20731. PMID 18780298. S2CID 13477741.
- Braitenberg, Valentino (1984). Vehicles-experiments in synthetic psychology. Cambridge, MA: MIT Press.
- Kinsbourne, Marcel (1978). "Evolution of language in relation to lateral action". Asymmetrical function of the brain. Cambridge: Cambridge University Press. pp. 553–565.
- Keller, Philipp J.; Schmidt, Annette D.; Wittbrodt, Joachim; Stelzer, Ernst H. K. (2008). "Reconstruction of zebrafish early embryonic development by scanned light sheet microscopy". Science. 322 (5904): 1065–1069. Bibcode:2008Sci...322.1065K. doi:10.1126/science.1162493. PMID 18845710. S2CID 7594561.
- de Lussanet, Marc H. E. (8 September 2023). Movie of an embryological twist in zebra fish embryo as revealed by cellular movement patterns (Quicktime Movie). Figshare. doi:10.6084/m9.figshare.24104085.
- Hamburger, Viktor; Hamilton, Howard L. (1951). "A series of normal stages in the development of the chick embryo". Dev. Dyn. 88 (4): 49–92. doi:10.1002/aja.1001950404. PMID 1304821. S2CID 32593520.
- Lopez-Sanchez, Carmen; Garcia-Martinez, Virginio; Schoenwolf, Gary C. (2001). "Localization of cells of the prospective neural plate, heart and somites within the primitive streak and epiblast of avian embryos at intermediate primitive-streak stages". Cells Tissues Organs. 169 (4): 334–346. doi:10.1159/000047900. PMID 11490112. S2CID 238761.
- Bellairs, Ruth; Osmond, Mark (1956). The atlas of chick development (2 ed.). New York: Academic Press. p. 172. ISBN 9780123849526.
- Pieper, Tobias; Carpaij, Meriam; Reinermann, Johanna; Surchev, Lachezar; Viebahn, Christoph; Tsikolia, Nikoloz (2020). "Matrix-filled microcavities in the emerging avian left-right organizer". Dev. Dyn. 249 (4): 496–508. doi:10.1002/dvdy.133. PMID 31729123. S2CID 208037861.
- Tsikolia, N; Schröder, S; Schwartz, P; Viebahn, C. (2012). "Paraxial left-sided nodal expression and the start of left-right patterning in the early chick embryo". Differentiation. 84 (5): 380–391. doi:10.1016/j.diff.2012.09.001. PMID 23142734.
- Isaac, Alison; Sargent, Michael G.; Cooke, Jonathan (1997). "Control of Vertebrate Left-Right Asymmetry by a Snail-Related Zinc Finger Gene". Science. 275 (5304): 1301–1304. doi:10.1126/science.275.5304.1301. PMID 9036854. S2CID 19753550.
- Toga, A. W.; Thompson, P. M. (2003). "Mapping brain asymmetry". Nat. Rev. Neurosci. 4 (1): 37–48. doi:10.1038/nrn1009. PMID 12511860. S2CID 15867592.
- Viggiano, D.; Pirolo, L. (2002). "Testing the model of optic chiasm formation in human beings". Brain Res. Bull. 59 (2): 111–115. doi:10.1016/S0361-9230(02)00846-8. PMID 12379441. S2CID 2687785.
- Nieuwenhuys, R.; Donkelaar, H. J.; Nicholson, C.; Smeets, W. J. A. J.; Wicht, H. (1998). The central nervous system of vertebrates. New York: Springer. ISBN 9783642621277.
- Marinelli, Wilhelm; Strenger, Anneliese (1956). Vergleichende Anatomie und Morphologie der Wirbeltiere, 1. Lampetra fluviatilis (L.) 2. Myxine glutinosa (L.) (in German). Vienna: Franz Deuticke. p. 172.
- Janvier, P. (1996). Early vertebrates. New York: Clarendon Press, Oxford University Press. ISBN 978-0198540472.
- Toga, Arthur W.; Thompson, Paul M. (2003). "Mapping brain asymmetry". Nat. Rev. Neurosci. 4 (1): 37–48. doi:10.1038/nrn1009. PMID 12511860. S2CID 15867592.
- Kouwenhoven, Jan-Willem; Vincken, Koen L.; Bartels, Lambertus W.; Castelein, Rene M. (2006). "Analysis of preexistent vertebral rotation in the normal spine". Spine. 31 (13): 1467–1472. doi:10.1097/01.brs.0000219938.14686.b3. PMID 16741456. S2CID 2401041.
- Mock, Jeffrey; Zadina, Janet; Corey, David; Cohen, Jeremy; Lemen, Lisa; Foundas, Anne (2012). "Atypical Brain Torque in Boys With Developmental Stuttering". Developmental Neuropsychology. 37 (5): 434–452. doi:10.1080/87565641.2012.661816. PMC 5537737. PMID 22799762.
- Maller, Jerome J.; Anderson, Rodney; Thomson, Richard H.; Rosenfeld, Jeffrey V.; Daskalakis, Zafiris J.; Fitzgerald, Paul B. (2015). "Occipital bending (Yakovlevian torque) in bipolar depression". Psychiatry Research: Neuroimaging. 231 (1): 8–14. doi:10.1016/j.pscychresns.2014.11.008. ISSN 0925-4927. PMID 25480522. S2CID 37224862.
- Nieuwenhuys, Rudolf; Voogd, Jan; van Huijzen, C. (2008). The human central nervous system (4th ed.). Berlin: Springer.
- Luiten, P. G. M. (1981). "Two visual pathways to the telencephalon in the nurse shark (Ginglymostoma cirratum). I. retinal projections". J. Comp. Neurol. 96 (4): 531–538. doi:10.1002/cne.901960402. PMID 7204669. S2CID 36572723.
- Luiten, P. G. M. (1981). "Two visual pathways to the telencephalon in the nurse shark (Ginglymostoma cirratum). II. ascending thalamo-telencephalic connections". J. Comp. Neurol. 96 (4): 539–548. doi:10.1002/cne.901960403. PMID 7204670. S2CID 13333558.
- Blanz, V.; Vetter, T. (1999). "A morphable model for the synthesis of 3D faces". SIGGRAPH'99 Conference Proceedings. 7: 187–194. doi:10.1145/311535.311556. hdl:11858/00-001M-0000-0013-E751-6. ISBN 0201485605. S2CID 207637109.
- Troje, N. F.; Bülthoff, H. H. (1996). "Face recognition under varying poses: The role of texture and shape". Vis. Res. 36 (12): 1761–1771. doi:10.1016/0042-6989(95)00230-8. PMID 8759445. S2CID 13115909.
- Kidokoro, Hinako; Saijoh, Yukio; Schoenwolf, Gary C. (2022). "Nodal signaling regulates asymmetric cellular behaviors, driving clockwise rotation of the heart tube in zebrafish". Communications Biology. 5 (1): 996. doi:10.1038/s42003-022-03826-7. PMC 9492702. PMID 36131094.
- "Why is the heart not in the middle of the body?". biology.stackexchange.com. Stack Overflow. 30 June 2017. Retrieved 12 September 2023.
- Geoffroy-Saint-Hilaire, Étienne (1822). "Considérations générales sur la vertèbre". Mémoires du Muséum d'Histoire Naturelle (in French). 9: 89–119.
- Dixon, A. Francis (1907). "Why are the great motor and sensory tracts of the central nervous system crossed?". The Dublin Journal of Medical Science. 124 (1): 1–4. doi:10.1007/BF02972358. S2CID 76086581.
- Nübler-Jung, Katharina; Arendt, Detlev (1996). "Enteropneusts and chordate evolution". Current Biology. 6 (4): 352–353. doi:10.1016/S0960-9822(02)00491-8. PMID 8723329. S2CID 8964529.
- Arendt, Detlev; Nübler-Jung, Katharina (1994). "Inversion of dorsoventral axis?". Nature. 371 (6492): 26. Bibcode:1994Natur.371...26A. doi:10.1038/371026a0. PMID 8072524. S2CID 33780610.
- de Lussanet, Marc H. E. (2011). "A hexamer origin of the echinoderms' five rays [on arXiv.org: with corrections]". Evol. Dev. 13 (2): 228–238. arXiv:1107.2223v2. doi:10.1111/j.1525-142x.2011.00472.x. PMID 21410878. S2CID 1938239.