Copernicium
Copernicium is a synthetic chemical element with the symbol Cn and atomic number 112. Its known isotopes are extremely radioactive, and have only been created in a laboratory. The most stable known isotope, copernicium-285, has a half-life of approximately 28 seconds. Copernicium was first created in 1996 by the GSI Helmholtz Centre for Heavy Ion Research near Darmstadt, Germany. It was named after the astronomer Nicolaus Copernicus.
Copernicium | |||||||||||||||||||||||||||||||||||||||||||||||
---|---|---|---|---|---|---|---|---|---|---|---|---|---|---|---|---|---|---|---|---|---|---|---|---|---|---|---|---|---|---|---|---|---|---|---|---|---|---|---|---|---|---|---|---|---|---|---|
Pronunciation | /ˌkoʊpərˈnɪsiəm/ | ||||||||||||||||||||||||||||||||||||||||||||||
Mass number | [285] | ||||||||||||||||||||||||||||||||||||||||||||||
Copernicium in the periodic table | |||||||||||||||||||||||||||||||||||||||||||||||
| |||||||||||||||||||||||||||||||||||||||||||||||
Atomic number (Z) | 112 | ||||||||||||||||||||||||||||||||||||||||||||||
Group | group 12 | ||||||||||||||||||||||||||||||||||||||||||||||
Period | period 7 | ||||||||||||||||||||||||||||||||||||||||||||||
Block | d-block | ||||||||||||||||||||||||||||||||||||||||||||||
Electron configuration | [Rn] 5f14 6d10 7s2 (predicted)[1] | ||||||||||||||||||||||||||||||||||||||||||||||
Electrons per shell | 2, 8, 18, 32, 32, 18, 2 (predicted) | ||||||||||||||||||||||||||||||||||||||||||||||
Physical properties | |||||||||||||||||||||||||||||||||||||||||||||||
Phase at STP | liquid (predicted)[2] | ||||||||||||||||||||||||||||||||||||||||||||||
Melting point | 283 ± 11 K (10 ± 11 °C, 50 ± 20 °F) (predicted)[2] | ||||||||||||||||||||||||||||||||||||||||||||||
Boiling point | 340 ± 10 K (67 ± 10 °C, 153 ± 18 °F)[2] (predicted) | ||||||||||||||||||||||||||||||||||||||||||||||
Density (near r.t.) | 14.0 g/cm3 (predicted)[2] | ||||||||||||||||||||||||||||||||||||||||||||||
Triple point | 283 K, 25 kPa (predicted)[2] | ||||||||||||||||||||||||||||||||||||||||||||||
Atomic properties | |||||||||||||||||||||||||||||||||||||||||||||||
Oxidation states | 0, (+1), +2, (+4), (+6) (parenthesized: prediction)[1][3][4][5] | ||||||||||||||||||||||||||||||||||||||||||||||
Ionization energies |
| ||||||||||||||||||||||||||||||||||||||||||||||
Atomic radius | calculated: 147 pm[1][4] (predicted) | ||||||||||||||||||||||||||||||||||||||||||||||
Covalent radius | 122 pm (predicted)[6] | ||||||||||||||||||||||||||||||||||||||||||||||
Other properties | |||||||||||||||||||||||||||||||||||||||||||||||
Natural occurrence | synthetic | ||||||||||||||||||||||||||||||||||||||||||||||
Crystal structure | hexagonal close-packed (hcp)![]() (predicted)[2] | ||||||||||||||||||||||||||||||||||||||||||||||
CAS Number | 54084-26-3 | ||||||||||||||||||||||||||||||||||||||||||||||
History | |||||||||||||||||||||||||||||||||||||||||||||||
Naming | after Nicolaus Copernicus | ||||||||||||||||||||||||||||||||||||||||||||||
Discovery | Gesellschaft für Schwerionenforschung (1996) | ||||||||||||||||||||||||||||||||||||||||||||||
Main isotopes of copernicium | |||||||||||||||||||||||||||||||||||||||||||||||
| |||||||||||||||||||||||||||||||||||||||||||||||
In the periodic table of the elements, copernicium is a d-block transactinide element and a group 12 element. During reactions with gold, it has been shown[10] to be an extremely volatile element, so much so that it is possibly a gas or a volatile liquid at standard temperature and pressure.
Copernicium is calculated to have several properties that differ from its lighter homologues in group 12, zinc, cadmium and mercury; due to relativistic effects, it may give up its 6d electrons instead of its 7s ones, and it may have more similarities to the noble gases such as radon rather than its group 12 homologues. Calculations indicate that copernicium may show the oxidation state +4, while mercury shows it in only one compound of disputed existence and zinc and cadmium do not show it at all. It has also been predicted to be more difficult to oxidize copernicium from its neutral state than the other group 12 elements. Predictions vary on whether solid copernicium would be a metal, semiconductor, or insulator. Copernicium is one of the heaviest elements whose chemical properties have been experimentally investigated.
Introduction
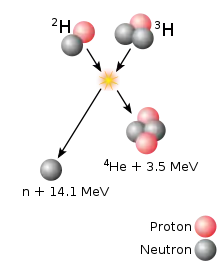
External video | |
---|---|
![]() |
The heaviest[lower-alpha 1] atomic nuclei are created in nuclear reactions that combine two other nuclei of unequal size[lower-alpha 2] into one; roughly, the more unequal the two nuclei in terms of mass, the greater the possibility that the two react.[17] The material made of the heavier nuclei is made into a target, which is then bombarded by the beam of lighter nuclei. Two nuclei can only fuse into one if they approach each other closely enough; normally, nuclei (all positively charged) repel each other due to electrostatic repulsion. The strong interaction can overcome this repulsion but only within a very short distance from a nucleus; beam nuclei are thus greatly accelerated in order to make such repulsion insignificant compared to the velocity of the beam nucleus.[18] Coming close alone is not enough for two nuclei to fuse: when two nuclei approach each other, they usually remain together for approximately 10−20 seconds and then part ways (not necessarily in the same composition as before the reaction) rather than form a single nucleus.[18][19] If fusion does occur, the temporary merger—termed a compound nucleus—is an excited state. To lose its excitation energy and reach a more stable state, a compound nucleus either fissions or ejects one or several neutrons,[lower-alpha 3] which carry away the energy. This occurs in approximately 10−16 seconds after the initial collision.[20][lower-alpha 4]
The beam passes through the target and reaches the next chamber, the separator; if a new nucleus is produced, it is carried with this beam.[23] In the separator, the newly produced nucleus is separated from other nuclides (that of the original beam and any other reaction products)[lower-alpha 5] and transferred to a surface-barrier detector, which stops the nucleus. The exact location of the upcoming impact on the detector is marked; also marked are its energy and the time of the arrival.[23] The transfer takes about 10−6 seconds; in order to be detected, the nucleus must survive this long.[26] The nucleus is recorded again once its decay is registered, and the location, the energy, and the time of the decay are measured.[23]
Stability of a nucleus is provided by the strong interaction. However, its range is very short; as nuclei become larger, their influence on the outermost nucleons (protons and neutrons) weakens. At the same time, the nucleus is torn apart by electrostatic repulsion between protons, as it has unlimited range.[27] Nuclei of the heaviest elements are thus theoretically predicted[28] and have so far been observed[29] to primarily decay via decay modes that are caused by such repulsion: alpha decay and spontaneous fission;[lower-alpha 6] these modes are predominant for nuclei of superheavy elements. Alpha decays are registered by the emitted alpha particles, and the decay products are easy to determine before the actual decay; if such a decay or a series of consecutive decays produces a known nucleus, the original product of a reaction can be determined arithmetically.[lower-alpha 7] Spontaneous fission, however, produces various nuclei as products, so the original nuclide cannot be determined from its daughters.[lower-alpha 8]
The information available to physicists aiming to synthesize one of the heaviest elements is thus the information collected at the detectors: location, energy, and time of arrival of a particle to the detector, and those of its decay. The physicists analyze this data and seek to conclude that it was indeed caused by a new element and could not have been caused by a different nuclide than the one claimed. Often, provided data is insufficient for a conclusion that a new element was definitely created and there is no other explanation for the observed effects; errors in interpreting data have been made.[lower-alpha 9]
History
Discovery
Copernicium was first created on February 9, 1996, at the Gesellschaft für Schwerionenforschung (GSI) in Darmstadt, Germany, by Sigurd Hofmann, Victor Ninov et al.[41] This element was created by firing accelerated zinc-70 nuclei at a target made of lead-208 nuclei in a heavy ion accelerator. A single atom of copernicium was produced with a mass number of 277. (A second was originally reported, but was found to have been based on data fabricated by Ninov, and was thus retracted.)[41]
- 208
82Pb + 70
30Zn → 278
112Cn* → 277
112Cn + 1
0n
In May 2000, the GSI successfully repeated the experiment to synthesize a further atom of copernicium-277.[42] This reaction was repeated at RIKEN using the Search for a Super-Heavy Element Using a Gas-Filled Recoil Separator set-up in 2004 and 2013 to synthesize three further atoms and confirm the decay data reported by the GSI team.[43][44] This reaction had also previously been tried in 1971 at the Joint Institute for Nuclear Research in Dubna, Russia to aim for 276Cn (produced in the 2n channel), but without success.[45]
The IUPAC/IUPAP Joint Working Party (JWP) assessed the claim of copernicium's discovery by the GSI team in 2001[46] and 2003.[47] In both cases, they found that there was insufficient evidence to support their claim. This was primarily related to the contradicting decay data for the known nuclide rutherfordium-261. However, between 2001 and 2005, the GSI team studied the reaction 248Cm(26Mg,5n)269Hs, and were able to confirm the decay data for hassium-269 and rutherfordium-261. It was found that the existing data on rutherfordium-261 was for an isomer,[48] now designated rutherfordium-261m.
In May 2009, the JWP reported on the claims of discovery of element 112 again and officially recognized the GSI team as the discoverers of element 112.[49] This decision was based on the confirmation of the decay properties of daughter nuclei as well as the confirmatory experiments at RIKEN.[50]
Work had also been done at the Joint Institute for Nuclear Research in Dubna, Russia from 1998 to synthesise the heavier isotope 283Cn in the hot fusion reaction 238U(48Ca,3n)283Cn; most observed atoms of 283Cn decayed by spontaneous fission, although an alpha decay branch to 279Ds was detected. While initial experiments aimed to assign the produced nuclide with its observed long half-life of 3 minutes based on its chemical behaviour, this was found to be not mercury-like as would have been expected (copernicium being under mercury in the periodic table),[50] and indeed now it appears that the long-lived activity might not have been from 283Cn at all, but its electron capture daughter 283Rg instead, with a shorter 4-second half-life associated with 283Cn. (Another possibility is assignment to a metastable isomeric state, 283mCn.)[51] While later cross-bombardments in the 242Pu+48Ca and 245Cm+48Ca reactions succeeded in confirming the properties of 283Cn and its parents 287Fl and 291Lv, and played a major role in the acceptance of the discoveries of flerovium and livermorium (elements 114 and 116) by the JWP in 2011, this work originated subsequent to the GSI's work on 277Cn and priority was assigned to the GSI.[50]
Naming

Using Mendeleev's nomenclature for unnamed and undiscovered elements, copernicium should be known as eka-mercury. In 1979, IUPAC published recommendations according to which the element was to be called ununbium (with the corresponding symbol of Uub),[52] a systematic element name as a placeholder, until the element was discovered (and the discovery then confirmed) and a permanent name was decided on. Although widely used in the chemical community on all levels, from chemistry classrooms to advanced textbooks, the recommendations were mostly ignored among scientists in the field, who either called it "element 112", with the symbol of E112, (112), or even simply 112.[1]
After acknowledging the GSI team's discovery, the IUPAC asked them to suggest a permanent name for element 112.[50][53] On 14 July 2009, they proposed copernicium with the element symbol Cp, after Nicolaus Copernicus "to honor an outstanding scientist, who changed our view of the world".[54]
During the standard six-month discussion period among the scientific community about the naming,[55][56] it was pointed out that the symbol Cp was previously associated with the name cassiopeium (cassiopium), now known as lutetium (Lu), and the compound cyclopentadiene.[57][58] For this reason, the IUPAC disallowed the use of Cp as a future symbol, prompting the GSI team to put forward the symbol Cn as an alternative. On 19 February 2010, the 537th anniversary of Copernicus' birth, IUPAC officially accepted the proposed name and symbol.[55][59]
Isotopes
Isotope | Half-life[lower-alpha 10] | Decay mode |
Discovery year[60] |
Discovery reaction[61] | |
---|---|---|---|---|---|
Value | Ref | ||||
277Cn | 0.85 ms | [60] | α | 1996 | 208Pb(70Zn,n) |
281Cn | 0.18 s | [7] | α | 2010 | 285Fl(—,α) |
282Cn | 0.91 ms | [62] | SF | 2003 | 290Lv(—,2α) |
283Cn | 4.2 s | [62] | α, SF, EC? | 2003 | 287Fl(—,α) |
284Cn | 98 ms | [62] | α, SF | 2004 | 288Fl(—,α) |
285Cn | 28 s | [62] | α | 1999 | 289Fl(—,α) |
285mCn[lower-alpha 11] | 15 s | [60] | α | 2012 | 293mLv(—,2α) |
286Cn[lower-alpha 11] | 8.45 s | [63] | SF | 2016 | 294Lv(—,2α) |
Copernicium has no stable or naturally occurring isotopes. Several radioactive isotopes have been synthesized in the laboratory, either by fusing two atoms or by observing the decay of heavier elements. Seven different isotopes have been reported with mass numbers 277 and 281–286, and one unconfirmed metastable isomer in 285Cn has been reported.[64] Most of these decay predominantly through alpha decay, but some undergo spontaneous fission, and copernicium-283 may have an electron capture branch.[65]
The isotope copernicium-283 was instrumental in the confirmation of the discoveries of the elements flerovium and livermorium.[66]
Half-lives
All confirmed copernicium isotopes are extremely unstable and radioactive; in general, heavier isotopes are more stable than the lighter. The most stable known isotope, 285Cn, has a half-life of 29 seconds; 283Cn has a half-life of 4 seconds, and the unconfirmed 285mCn and 286Cn have half-lives of about 15 and 8.45 seconds respectively. Other isotopes have half-lives shorter than one second. 281Cn and 284Cn both have half-lives on the order of 0.1 seconds, and the other two isotopes have half-lives slightly under one millisecond.[65] It is predicted that the heavy isotopes 291Cn and 293Cn may have half-lives longer than a few decades, for they are predicted to lie near the center of the theoretical island of stability, and may have been produced in the r-process and be detectable in cosmic rays, though they would be about 10−12 times as abundant as lead.[67]
The lightest isotopes of copernicium have been synthesized by direct fusion between two lighter nuclei and as decay products (except for 277Cn, which is not known to be a decay product), while the heavier isotopes are only known to be produced by decay of heavier nuclei. The heaviest isotope produced by direct fusion is 283Cn; the three heavier isotopes, 284Cn, 285Cn, and 286Cn, have only been observed as decay products of elements with larger atomic numbers.[65]
In 1999, American scientists at the University of California, Berkeley, announced that they had succeeded in synthesizing three atoms of 293Og.[68] These parent nuclei were reported to have successively emitted three alpha particles to form copernicium-281 nuclei, which were claimed to have undergone alpha decay, emitting alpha particles with decay energy 10.68 MeV and half-life 0.90 ms, but their claim was retracted in 2001[69] as it had been based on data fabricated by Ninov.[70] This isotope was truly produced in 2010 by the same team; the new data contradicted the previous fabricated data.[71]
Predicted properties
Very few properties of copernicium or its compounds have been measured; this is due to its extremely limited and expensive production[72] and the fact that copernicium (and its parents) decays very quickly. A few singular chemical properties have been measured, as well as the boiling point, but properties of the copernicium metal remain generally unknown and for the most part, only predictions are available.
Chemical
Copernicium is the tenth and last member of the 6d series and is the heaviest group 12 element in the periodic table, below zinc, cadmium and mercury. It is predicted to differ significantly from the lighter group 12 elements. The valence s-subshells of the group 12 elements and period 7 elements are expected to be relativistically contracted most strongly at copernicium. This and the closed-shell configuration of copernicium result in it probably being a very noble metal. A standard reduction potential of +2.1 V is predicted for the Cn2+/Cn couple. Copernicium's predicted first ionization energy of 1155 kJ/mol almost matches that of the noble gas xenon at 1170.4 kJ/mol.[1] Copernicium's metallic bonds should also be very weak, possibly making it extremely volatile like the noble gases, and potentially making it gaseous at room temperature.[1][73] However, it should be able to form metal–metal bonds with copper, palladium, platinum, silver, and gold; these bonds are predicted to be only about 15–20 kJ/mol weaker than the analogous bonds with mercury.[1] In opposition to the earlier suggestion,[74] ab initio calculations at the high level of accuracy[75] predicted that the chemistry of singly-valent copernicium resembles that of mercury rather than that of the noble gases. The latter result can be explained by the huge spin-orbit interaction which significantly lowers the energy of the vacant 7p1/2 state of copernicium.
Once copernicium is ionized, its chemistry may present several differences from those of zinc, cadmium, and mercury. Due to the stabilization of 7s electronic orbitals and destabilization of 6d ones caused by relativistic effects, Cn2+ is likely to have a [Rn]5f146d87s2 electronic configuration, using the 6d orbitals before the 7s one, unlike its homologues. The fact that the 6d electrons participate more readily in chemical bonding means that once copernicium is ionized, it may behave more like a transition metal than its lighter homologues, especially in the possible +4 oxidation state. In aqueous solutions, copernicium may form the +2 and perhaps +4 oxidation states.[1] The diatomic ion Hg2+
2, featuring mercury in the +1 oxidation state, is well-known, but the Cn2+
2 ion is predicted to be unstable or even non-existent.[1] Copernicium(II) fluoride, CnF2, should be more unstable than the analogous mercury compound, mercury(II) fluoride (HgF2), and may even decompose spontaneously into its constituent elements. As the most electronegative reactive element, fluorine may be the only element able to oxidise copernicium even further to the +4 and even +6 oxidation states in CnF4 and CnF6; the latter may require matrix-isolation conditions to be detected, as in the disputed detection of HgF4. CnF4 should be more stable than CnF2.[5] In polar solvents, copernicium is predicted to preferentially form the CnF−
5 and CnF−
3 anions rather than the analogous neutral fluorides (CnF4 and CnF2, respectively), although the analogous bromide or iodide ions may be more stable towards hydrolysis in aqueous solution. The anions CnCl2−
4 and CnBr2−
4 should also be able to exist in aqueous solution.[1] The formation of thermodynamically stable copernicium(II) and (IV) fluorides would be analogous to the chemistry of xenon.[2] Analogous to mercury(II) cyanide (Hg(CN)2), copernicium is expected to form a stable cyanide, Cn(CN)2.[76]
Physical and atomic
Copernicium should be a dense metal, with a density of 14.0 g/cm3 in the liquid state at 300 K; this is similar to the known density of mercury, which is 13.534 g/cm3. (Solid copernicium at the same temperature should have a higher density of 14.7 g/cm3.) This results from the effects of copernicium's higher atomic weight being cancelled out by its larger interatomic distances compared to mercury.[2] Some calculations predicted copernicium to be a gas at room temperature due to its closed-shell electron configuration,[77] which would make it the first gaseous metal in the periodic table.[1][73] A 2019 calculation agrees with these predictions on the role of relativistic effects, suggesting that copernicium will be a volatile liquid bound by dispersion forces under standard conditions. Its melting point is estimated at 283±11 K and its boiling point at 340±10 K, the latter in agreement with the experimentally estimated value of 357+112
−108 K.[2] The atomic radius of copernicium is expected to be around 147 pm. Due to the relativistic stabilization of the 7s orbital and destabilization of the 6d orbital, the Cn+ and Cn2+ ions are predicted to give up 6d electrons instead of 7s electrons, which is the opposite of the behavior of its lighter homologues.[1]
In addition to the relativistic contraction and binding of the 7s subshell, the 6d5/2 orbital is expected to be destabilized due to spin-orbit coupling, making it behave similarly to the 7s orbital in terms of size, shape, and energy. Predictions of the expected band structure of copernicium are varied. Calculations in 2007 expected that copernicium may be a semiconductor[78] with a band gap of around 0.2 eV,[79] crystallizing in the hexagonal close-packed crystal structure.[79] However, calculations in 2017 and 2018 suggested that copernicium should be a noble metal at standard conditions with a body-centered cubic crystal structure: it should hence have no band gap, like mercury, although the density of states at the Fermi level is expected to be lower for copernicium than for mercury.[80][81] 2019 calculations then suggested that in fact copernicium has a large band gap of 6.4 ± 0.2 eV, which should be similar to that of the noble gas radon (predicted as 7.1 eV) and would make it an insulator; bulk copernicium is predicted by these calculations to be bound mostly by dispersion forces, like the noble gases.[2] Like mercury, radon, and flerovium, but not oganesson (eka-radon), copernicium is calculated to have no electron affinity.[82]
Experimental atomic gas phase chemistry
Interest in copernicium's chemistry was sparked by predictions that it would have the largest relativistic effects in the whole of period 7 and group 12, and indeed among all 118 known elements.[1] Copernicium is expected to have the ground state electron configuration [Rn] 5f14 6d10 7s2 and thus should belong to group 12 of the periodic table, according to the Aufbau principle. As such, it should behave as the heavier homologue of mercury and form strong binary compounds with noble metals like gold. Experiments probing the reactivity of copernicium have focused on the adsorption of atoms of element 112 onto a gold surface held at varying temperatures, in order to calculate an adsorption enthalpy. Owing to relativistic stabilization of the 7s electrons, copernicium shows radon-like properties. Experiments were performed with the simultaneous formation of mercury and radon radioisotopes, allowing a comparison of adsorption characteristics.[83]
The first chemical experiments on copernicium were conducted using the 238U(48Ca,3n)283Cn reaction. Detection was by spontaneous fission of the claimed parent isotope with half-life of 5 minutes. Analysis of the data indicated that copernicium was more volatile than mercury and had noble gas properties. However, the confusion regarding the synthesis of copernicium-283 has cast some doubt on these experimental results.[83] Given this uncertainty, between April–May 2006 at the JINR, a FLNR–PSI team conducted experiments probing the synthesis of this isotope as a daughter in the nuclear reaction 242Pu(48Ca,3n)287Fl.[83] (The 242Pu + 48Ca fusion reaction has a slightly larger cross-section than the 238U + 48Ca reaction, so that the best way to produce copernicium for chemical experimentation is as an overshoot product as the daughter of flerovium.)[84] In this experiment, two atoms of copernicium-283 were unambiguously identified and the adsorption properties were interpret to show that copernicium is a more volatile homologue of mercury, due to formation of a weak metal-metal bond with gold.[83] This agrees with general indications from some relativistic calculations that copernicium is "more or less" homologous to mercury.[85] However, it was pointed out in 2019 that this result may simply be due to strong dispersion interactions.[2]
In April 2007, this experiment was repeated and a further three atoms of copernicium-283 were positively identified. The adsorption property was confirmed and indicated that copernicium has adsorption properties in agreement with being the heaviest member of group 12.[83] These experiments also allowed the first experimental estimation of copernicium's boiling point: 84+112
−108 °C, so that it may be a gas at standard conditions.[78]
Because the lighter group 12 elements often occur as chalcogenide ores, experiments were conducted in 2015 to deposit copernicium atoms on a selenium surface to form copernicium selenide, CnSe. Reaction of copernicium atoms with trigonal selenium to form a selenide was observed, with -ΔHadsCn(t-Se) > 48 kJ/mol, with the kinetic hindrance towards selenide formation being lower for copernicium than for mercury. This was unexpected as the stability of the group 12 selenides tends to decrease down the group from ZnSe to HgSe.[86]
See also
- Island of stability
Notes
- In nuclear physics, an element is called heavy if its atomic number is high; lead (element 82) is one example of such a heavy element. The term "superheavy elements" typically refers to elements with atomic number greater than 103 (although there are other definitions, such as atomic number greater than 100[12] or 112;[13] sometimes, the term is presented an equivalent to the term "transactinide", which puts an upper limit before the beginning of the hypothetical superactinide series).[14] Terms "heavy isotopes" (of a given element) and "heavy nuclei" mean what could be understood in the common language—isotopes of high mass (for the given element) and nuclei of high mass, respectively.
- In 2009, a team at JINR led by Oganessian published results of their attempt to create hassium in a symmetric 136Xe + 136Xe reaction. They failed to observe a single atom in such a reaction, putting the upper limit on the cross section, the measure of probability of a nuclear reaction, as 2.5 pb.[15] In comparison, the reaction that resulted in hassium discovery, 208Pb + 58Fe, had a cross section of ~20 pb (more specifically, 19+19
−11 pb), as estimated by the discoverers.[16] - The greater the excitation energy, the more neutrons are ejected. If the excitation energy is lower than energy binding each neutron to the rest of the nucleus, neutrons are not emitted; instead, the compound nucleus de-excites by emitting a gamma ray.[20]
- The definition by the IUPAC/IUPAP Joint Working Party states that a chemical element can only be recognized as discovered if a nucleus of it has not decayed within 10−14 seconds. This value was chosen as an estimate of how long it takes a nucleus to acquire its outer electrons and thus display its chemical properties.[21] This figure also marks the generally accepted upper limit for lifetime of a compound nucleus.[22]
- This separation is based on that the resulting nuclei move past the target more slowly then the unreacted beam nuclei. The separator contains electric and magnetic fields whose effects on a moving particle cancel out for a specific velocity of a particle.[24] Such separation can also be aided by a time-of-flight measurement and a recoil energy measurement; a combination of the two may allow to estimate the mass of a nucleus.[25]
- Not all decay modes are caused by electrostatic repulsion. For example, beta decay is caused by the weak interaction.[30]
- Since mass of a nucleus is not measured directly but is rather calculated from that of another nucleus, such measurement is called indirect. Direct measurements are also possible, but for the most part they have remained unavailable for heaviest nuclei.[31] The first direct measurement of mass of a superheavy nucleus was reported in 2018 at LBNL.[32] Mass was determined from the location of a nucleus after the transfer (the location helps determine its trajectory, which is linked to the mass-to-charge ratio of the nucleus, since the transfer was done in presence of a magnet).[33]
- Spontaneous fission was discovered by Soviet physicist Georgy Flerov,[34] a leading scientist at JINR, and thus it was a "hobbyhorse" for the facility.[35] In contrast, the LBL scientists believed fission information was not sufficient for a claim of synthesis of an element. They believed spontaneous fission had not been studied enough to use it for identification of a new element, since there was a difficulty of establishing that a compound nucleus had only ejected neutrons and not charged particles like protons or alpha particles.[22] They thus preferred to link new isotopes to the already known ones by successive alpha decays.[34]
- For instance, element 102 was mistakenly identified in 1957 at the Nobel Institute of Physics in Stockholm, Stockholm County, Sweden.[36] There were no earlier definitive claims of creation of this element, and the element was assigned a name by its Swedish, American, and British discoverers, nobelium. It was later shown that the identification was incorrect.[37] The following year, LBNL was unable to reproduce the Swedish results and announced instead their synthesis of the element; that claim was also disproved later.[37] JINR insisted that they were the first to create the element and suggested a name of their own for the new element, joliotium;[38] the Soviet name was also not accepted (JINR later referred to the naming of element 102 as "hasty").[39] The name "nobelium" remained unchanged on account of its widespread usage.[40]
- Different sources give different values for half-lives; the most recently published values are listed.
- This isotope is unconfirmed
References
- Hoffman, Darleane C.; Lee, Diana M.; Pershina, Valeria (2006). "Transactinides and the future elements". In Morss; Edelstein, Norman M.; Fuger, Jean (eds.). The Chemistry of the Actinide and Transactinide Elements (3rd ed.). Dordrecht, The Netherlands: Springer Science+Business Media. ISBN 978-1-4020-3555-5.
- Gäggeler, Heinz W.; Türler, Andreas (2013). "Gas Phase Chemistry of Superheavy Elements". The Chemistry of Superheavy Elements. Springer Science+Business Media. pp. 415–483. doi:10.1007/978-3-642-37466-1_8. ISBN 978-3-642-37465-4. Retrieved April 21, 2018.
- Fricke, Burkhard (1975). "Superheavy elements: a prediction of their chemical and physical properties". Recent Impact of Physics on Inorganic Chemistry. Structure and Bonding. 21: 89–144. doi:10.1007/BFb0116498. ISBN 978-3-540-07109-9. Retrieved October 4, 2013.
- Hu, Shu-Xian; Zou, Wenli (September 23, 2021). "Stable copernicium hexafluoride (CnF6) with an oxidation state of VI+". Physical Chemistry Chemical Physics. 2022 (24): 321–325. doi:10.1039/D1CP04360A.
- Chemical Data. Copernicium - Cn, Royal Chemical Society
- Utyonkov, V. K.; Brewer, N. T.; Oganessian, Yu. Ts.; et al. (January 30, 2018). "Neutron-deficient superheavy nuclei obtained in the 240Pu+48Ca reaction". Physical Review C. 97 (14320): 1–10. Bibcode:2018PhRvC..97a4320U. doi:10.1103/PhysRevC.97.014320.
- Chart of Nuclides. Brookhaven National Laboratory
- Såmark-Roth, A.; Cox, D. M.; Rudolph, D.; et al. (2021). "Spectroscopy along Flerovium Decay Chains: Discovery of 280Ds and an Excited State in 282Cn". Physical Review Letters. 126: 032503. doi:10.1103/PhysRevLett.126.032503.
- Eichler, R.; et al. (2007). "Chemical Characterization of Element 112". Nature. 447 (7140): 72–75. Bibcode:2007Natur.447...72E. doi:10.1038/nature05761. PMID 17476264. S2CID 4347419.
- Wakhle, A.; Simenel, C.; Hinde, D. J.; et al. (2015). Simenel, C.; Gomes, P. R. S.; Hinde, D. J.; et al. (eds.). "Comparing Experimental and Theoretical Quasifission Mass Angle Distributions". European Physical Journal Web of Conferences. 86: 00061. Bibcode:2015EPJWC..8600061W. doi:10.1051/epjconf/20158600061. ISSN 2100-014X.
- Krämer, K. (2016). "Explainer: superheavy elements". Chemistry World. Retrieved March 15, 2020.
- "Discovery of Elements 113 and 115". Lawrence Livermore National Laboratory. Archived from the original on September 11, 2015. Retrieved March 15, 2020.
- Eliav, E.; Kaldor, U.; Borschevsky, A. (2018). "Electronic Structure of the Transactinide Atoms". In Scott, R. A. (ed.). Encyclopedia of Inorganic and Bioinorganic Chemistry. John Wiley & Sons. pp. 1–16. doi:10.1002/9781119951438.eibc2632. ISBN 978-1-119-95143-8. S2CID 127060181.
- Oganessian, Yu. Ts.; Dmitriev, S. N.; Yeremin, A. V.; et al. (2009). "Attempt to produce the isotopes of element 108 in the fusion reaction 136Xe + 136Xe". Physical Review C. 79 (2): 024608. doi:10.1103/PhysRevC.79.024608. ISSN 0556-2813.
- Münzenberg, G.; Armbruster, P.; Folger, H.; et al. (1984). "The identification of element 108" (PDF). Zeitschrift für Physik A. 317 (2): 235–236. Bibcode:1984ZPhyA.317..235M. doi:10.1007/BF01421260. S2CID 123288075. Archived from the original (PDF) on June 7, 2015. Retrieved October 20, 2012.
- Subramanian, S. (2019). "Making New Elements Doesn't Pay. Just Ask This Berkeley Scientist". Bloomberg Businessweek. Archived from the original on November 14, 2020. Retrieved January 18, 2020.
- Ivanov, D. (2019). "Сверхтяжелые шаги в неизвестное" [Superheavy steps into the unknown]. N+1 (in Russian). Retrieved February 2, 2020.
- Hinde, D. (2014). "Something new and superheavy at the periodic table". The Conversation. Retrieved January 30, 2020.
- Krása, A. (2010). "Neutron Sources for ADS" (PDF). Czech Technical University in Prague. pp. 4–8. S2CID 28796927. Archived from the original (PDF) on March 3, 2019. Retrieved October 20, 2019.
- Wapstra, A. H. (1991). "Criteria that must be satisfied for the discovery of a new chemical element to be recognized" (PDF). Pure and Applied Chemistry. 63 (6): 883. doi:10.1351/pac199163060879. ISSN 1365-3075. S2CID 95737691. Retrieved August 28, 2020.
- Hyde, E. K.; Hoffman, D. C.; Keller, O. L. (1987). "A History and Analysis of the Discovery of Elements 104 and 105". Radiochimica Acta. 42 (2): 67–68. doi:10.1524/ract.1987.42.2.57. ISSN 2193-3405. S2CID 99193729.
- Chemistry World (2016). "How to Make Superheavy Elements and Finish the Periodic Table [Video]". Scientific American. Retrieved January 27, 2020.
- Hoffman, Ghiorso & Seaborg 2000, p. 334.
- Hoffman, Ghiorso & Seaborg 2000, p. 335.
- Zagrebaev, Karpov & Greiner 2013, p. 3.
- Beiser 2003, p. 432.
- Staszczak, A.; Baran, A.; Nazarewicz, W. (2013). "Spontaneous fission modes and lifetimes of superheavy elements in the nuclear density functional theory". Physical Review C. 87 (2): 024320–1. arXiv:1208.1215. Bibcode:2013PhRvC..87b4320S. doi:10.1103/physrevc.87.024320. ISSN 0556-2813. S2CID 118134429.
- Audi et al. 2017, pp. 030001-128–030001-138.
- Beiser 2003, p. 439.
- Oganessian, Yu. Ts.; Rykaczewski, K. P. (2015). "A beachhead on the island of stability". Physics Today. 68 (8): 32–38. Bibcode:2015PhT....68h..32O. doi:10.1063/PT.3.2880. ISSN 0031-9228. OSTI 1337838. S2CID 119531411.
- Grant, A. (2018). "Weighing the heaviest elements". Physics Today. doi:10.1063/PT.6.1.20181113a. S2CID 239775403.
- Howes, L. (2019). "Exploring the superheavy elements at the end of the periodic table". Chemical & Engineering News. Retrieved January 27, 2020.
- Robinson, A. E. (2019). "The Transfermium Wars: Scientific Brawling and Name-Calling during the Cold War". Distillations. Retrieved February 22, 2020.
- "Популярная библиотека химических элементов. Сиборгий (экавольфрам)" [Popular library of chemical elements. Seaborgium (eka-tungsten)]. n-t.ru (in Russian). Retrieved January 7, 2020. Reprinted from "Экавольфрам" [Eka-tungsten]. Популярная библиотека химических элементов. Серебро — Нильсборий и далее [Popular library of chemical elements. Silver through nielsbohrium and beyond] (in Russian). Nauka. 1977.
- "Nobelium – Element information, properties and uses | Periodic Table". Royal Society of Chemistry. Retrieved March 1, 2020.
- Kragh 2018, pp. 38–39.
- Kragh 2018, p. 40.
- Ghiorso, A.; Seaborg, G. T.; Oganessian, Yu. Ts.; et al. (1993). "Responses on the report 'Discovery of the Transfermium elements' followed by reply to the responses by Transfermium Working Group" (PDF). Pure and Applied Chemistry. 65 (8): 1815–1824. doi:10.1351/pac199365081815. S2CID 95069384. Archived (PDF) from the original on November 25, 2013. Retrieved September 7, 2016.
- Commission on Nomenclature of Inorganic Chemistry (1997). "Names and symbols of transfermium elements (IUPAC Recommendations 1997)" (PDF). Pure and Applied Chemistry. 69 (12): 2471–2474. doi:10.1351/pac199769122471.
- Hofmann, S.; et al. (1996). "The new element 112". Zeitschrift für Physik A. 354 (1): 229–230. Bibcode:1996ZPhyA.354..229H. doi:10.1007/BF02769517. S2CID 119975957.
- Hofmann, S.; et al. (2000). "New Results on Element 111 and 112" (PDF). European Physical Journal A. Gesellschaft für Schwerionenforschung. 14 (2): 147–157. Bibcode:2002EPJA...14..147H. doi:10.1140/epja/i2001-10119-x. S2CID 8773326. Archived from the original (PDF) on February 27, 2008. Retrieved March 2, 2008.
- Morita, K. (2004). "Decay of an Isotope 277112 produced by 208Pb + 70Zn reaction". In Penionzhkevich, Yu. E.; Cherepanov, E. A. (eds.). Exotic Nuclei: Proceedings of the International Symposium. World Scientific. pp. 188–191. doi:10.1142/9789812701749_0027.
- Sumita, Takayuki; Morimoto, Kouji; Kaji, Daiya; Haba, Hiromitsu; Ozeki, Kazutaka; Sakai, Ryutaro; Yoneda, Akira; Yoshida, Atsushi; Hasebe, Hiroo; Katori, Kenji; Sato, Nozomi; Wakabayashi, Yasuo; Mitsuoka, Shin-Ichi; Goto, Shin-Ichi; Murakami, Masashi; Kariya, Yoshiki; Tokanai, Fuyuki; Mayama, Keita; Takeyama, Mirei; Moriya, Toru; Ideguchi, Eiji; Yamaguchi, Takayuki; Kikunaga, Hidetoshi; Chiba, Junsei; Morita, Kosuke (2013). "New Result on the Production of277Cn by the208Pb +70Zn Reaction". Journal of the Physical Society of Japan. 82 (2): 024202. Bibcode:2013JPSJ...82b4202S. doi:10.7566/JPSJ.82.024202.
- Popeko, Andrey G. (2016). "Synthesis of superheavy elements" (PDF). jinr.ru. Joint Institute for Nuclear Research. Archived from the original (PDF) on February 4, 2018. Retrieved February 4, 2018.
- Karol, P. J.; Nakahara, H.; Petley, B. W.; Vogt, E. (2001). "On the Discovery of the Elements 110–112" (PDF). Pure and Applied Chemistry. 73 (6): 959–967. doi:10.1351/pac200173060959. S2CID 97615948. Archived from the original (PDF) on March 9, 2018. Retrieved January 9, 2008.
- Karol, P. J.; Nakahara, H.; Petley, B. W.; Vogt, E. (2003). "On the Claims for Discovery of Elements 110, 111, 112, 114, 116 and 118" (PDF). Pure and Applied Chemistry. 75 (10): 1061–1611. doi:10.1351/pac200375101601. S2CID 95920517. Archived from the original (PDF) on August 22, 2016. Retrieved January 9, 2008.
- Dressler, R.; Türler, A. (2001). "Evidence for Isomeric States in 261Rf" (PDF). Annual Report. Paul Scherrer Institute. Archived from the original (PDF) on July 7, 2011.
- "A New Chemical Element in the Periodic Table". Gesellschaft für Schwerionenforschung. June 10, 2009. Archived from the original on August 23, 2009. Retrieved April 14, 2012.
- Barber, R. C.; et al. (2009). "Discovery of the element with atomic number 112" (PDF). Pure and Applied Chemistry. 81 (7): 1331. doi:10.1351/PAC-REP-08-03-05. S2CID 95703833.
- Hofmann, S.; Heinz, S.; Mann, R.; Maurer, J.; Münzenberg, G.; Antalic, S.; Barth, W.; Burkhard, H. G.; Dahl, L.; Eberhardt, K.; Grzywacz, R.; Hamilton, J. H.; Henderson, R. A.; Kenneally, J. M.; Kindler, B.; Kojouharov, I.; Lang, R.; Lommel, B.; Miernik, K.; Miller, D.; Moody, K. J.; Morita, K.; Nishio, K.; Popeko, A. G.; Roberto, J. B.; Runke, J.; Rykaczewski, K. P.; Saro, S.; Schneidenberger, C.; Schött, H. J.; Shaughnessy, D. A.; Stoyer, M. A.; Thörle-Pospiech, P.; Tinschert, K.; Trautmann, N.; Uusitalo, J.; Yeremin, A. V. (2016). "Remarks on the Fission Barriers of SHN and Search for Element 120". In Peninozhkevich, Yu. E.; Sobolev, Yu. G. (eds.). Exotic Nuclei: EXON-2016 Proceedings of the International Symposium on Exotic Nuclei. Exotic Nuclei. pp. 155–164. ISBN 9789813226555.
- Chatt, J. (1979). "Recommendations for the naming of elements of atomic numbers greater than 100". Pure and Applied Chemistry. 51 (2): 381–384. doi:10.1351/pac197951020381.
- "New Chemical Element in the Periodic Table". Science Daily. June 11, 2009.
- "Element 112 shall be named "copernicium"". Gesellschaft für Schwerionenforschung. July 14, 2009. Archived from the original on July 18, 2009.
- "New element named 'copernicium'". BBC News. July 16, 2009. Retrieved February 22, 2010.
- "Start of the Name Approval Process for the Element of Atomic Number 112". IUPAC. July 20, 2009. Archived from the original on November 27, 2012. Retrieved April 14, 2012.
- Meija, Juris (2009). "The need for a fresh symbol to designate copernicium". Nature. 461 (7262): 341. Bibcode:2009Natur.461..341M. doi:10.1038/461341c. PMID 19759598.
- van der Krogt, P. "Lutetium". Elementymology & Elements Multidict. Retrieved February 22, 2010.
- "IUPAC Element 112 is Named Copernicium". IUPAC. February 19, 2010. Archived from the original on March 4, 2016. Retrieved April 13, 2012.
- Audi, G.; Kondev, F. G.; Wang, M.; Huang, W. J.; Naimi, S. (2017). "The NUBASE2016 evaluation of nuclear properties" (PDF). Chinese Physics C. 41 (3): 030001. Bibcode:2017ChPhC..41c0001A. doi:10.1088/1674-1137/41/3/030001.
- Thoennessen, M. (2016). The Discovery of Isotopes: A Complete Compilation. Springer. pp. 229, 234, 238. doi:10.1007/978-3-319-31763-2. ISBN 978-3-319-31761-8. LCCN 2016935977.
- Oganessian, Y.T. (2015). "Super-heavy element research". Reports on Progress in Physics. 78 (3): 036301. Bibcode:2015RPPh...78c6301O. doi:10.1088/0034-4885/78/3/036301. PMID 25746203. S2CID 37779526.
- Kaji, Daiya; Morita, Kosuke; Morimoto, Kouji; Haba, Hiromitsu; Asai, Masato; Fujita, Kunihiro; Gan, Zaiguo; Geissel, Hans; Hasebe, Hiroo; Hofmann, Sigurd; Huang, MingHui; Komori, Yukiko; Ma, Long; Maurer, Joachim; Murakami, Masashi; Takeyama, Mirei; Tokanai, Fuyuki; Tanaka, Taiki; Wakabayashi, Yasuo; Yamaguchi, Takayuki; Yamaki, Sayaka; Yoshida, Atsushi (2017). "Study of the Reaction 48Ca + 248Cm → 296Lv* at RIKEN-GARIS". Journal of the Physical Society of Japan. 86 (3): 034201–1–7. Bibcode:2017JPSJ...86c4201K. doi:10.7566/JPSJ.86.034201.
- Hofmann, S.; Heinz, S.; Mann, R.; Maurer, J.; Khuyagbaatar, J.; Ackermann, D.; Antalic, S.; Barth, W.; Block, M.; Burkhard, H. G.; Comas, V. F.; Dahl, L.; Eberhardt, K.; Gostic, J.; Henderson, R. A.; Heredia, J. A.; Heßberger, F. P.; Kenneally, J. M.; Kindler, B.; Kojouharov, I.; Kratz, J. V.; Lang, R.; Leino, M.; Lommel, B.; Moody, K. J.; Münzenberg, G.; Nelson, S. L.; Nishio, K.; Popeko, A. G.; et al. (2012). "The reaction 48Ca + 248Cm → 296116* studied at the GSI-SHIP". The European Physical Journal A. 48 (5): 62. Bibcode:2012EPJA...48...62H. doi:10.1140/epja/i2012-12062-1. S2CID 121930293.
- Holden, N. E. (2004). "Table of the Isotopes". In D. R. Lide (ed.). CRC Handbook of Chemistry and Physics (85th ed.). CRC Press. Section 11. ISBN 978-0-8493-0485-9.
- Barber, R. C.; et al. (2011). "Discovery of the elements with atomic numbers greater than or equal to 113" (PDF). Pure and Applied Chemistry. 83 (7): 5–7. doi:10.1351/PAC-REP-10-05-01. S2CID 98065999.
- Zagrebaev, Karpov & Greiner 2013, pp. 1–15.
-
Ninov, V.; et al. (1999). "Observation of Superheavy Nuclei Produced in the Reaction of 86
Kr
with 208
Pb
". Physical Review Letters. 83 (6): 1104–1107. Bibcode:1999PhRvL..83.1104N. doi:10.1103/PhysRevLett.83.1104. - Public Affairs Department (July 21, 2001). "Results of element 118 experiment retracted". Berkeley Lab. Archived from the original on January 29, 2008. Retrieved January 18, 2008.
- At Lawrence Berkeley, Physicists Say a Colleague Took Them for a Ride George Johnson, The New York Times, 15 October 2002
- Public Affairs Department (October 26, 2010). "Six New Isotopes of the Superheavy Elements Discovered: Moving Closer to Understanding the Island of Stability". Berkeley Lab. Retrieved April 25, 2011.
- Subramanian, S. "Making New Elements Doesn't Pay. Just Ask This Berkeley Scientist". Bloomberg Businessweek. Retrieved January 18, 2020.
- "Chemistry on the islands of stability", New Scientist, 11 September 1975, p. 574, ISSN 1032-1233
- Pitzer, K. S. (1975). "Are elements 112, 114, and 118 relatively inert gases?". The Journal of Chemical Physics. 63 (2): 1032–1033. doi:10.1063/1.431398.
- Mosyagin, N. S.; Isaev, T. A.; Titov, A. V. (2006). "Is E112 a relatively inert element? Benchmark relativistic correlation study of spectroscopic constants in E112H and its cation". The Journal of Chemical Physics. 124 (22): 224302. arXiv:physics/0508024. Bibcode:2006JChPh.124v4302M. doi:10.1063/1.2206189. PMID 16784269. S2CID 119339584.
- Demissie, Taye B.; Ruud, Kenneth (February 25, 2017). "Darmstadtium, roentgenium, and copernicium form strong bonds with cyanide". International Journal of Quantum Chemistry. 2017: e25393. doi:10.1002/qua.25393. hdl:10037/13632.
- Kratz, Jens Volker. The Impact of Superheavy Elements on the Chemical and Physical Sciences. 4th International Conference on the Chemistry and Physics of the Transactinide Elements, 5 – 11 September 2011, Sochi, Russia
- Eichler, R.; Aksenov, N. V.; Belozerov, A. V.; Bozhikov, G. A.; Chepigin, V. I.; Dmitriev, S. N.; Dressler, R.; Gäggeler, H. W.; et al. (2008). "Thermochemical and physical properties of element 112". Angewandte Chemie. 47 (17): 3262–6. doi:10.1002/anie.200705019. PMID 18338360.
- Gaston, Nicola; Opahle, Ingo; Gäggeler, Heinz W.; Schwerdtfeger, Peter (2007). "Is eka-mercury (element 112) a group 12 metal?". Angewandte Chemie. 46 (10): 1663–6. doi:10.1002/anie.200604262. PMID 17397075. Retrieved November 5, 2013.
- Gyanchandani, Jyoti; Mishra, Vinayak; Dey, G. K.; Sikka, S. K. (January 2018). "Super heavy element Copernicium: Cohesive and electronic properties revisited". Solid State Communications. 269: 16–22. Bibcode:2018SSCom.269...16G. doi:10.1016/j.ssc.2017.10.009. Retrieved March 28, 2018.
- Čenčariková, Hana; Legut, Dominik (2018). "The effect of relativity on stability of Copernicium phases, their electronic structure and mechanical properties". Physica B. 536: 576–582. arXiv:1810.01955. Bibcode:2018PhyB..536..576C. doi:10.1016/j.physb.2017.11.035. S2CID 119100368.
- Borschevsky, Anastasia; Pershina, Valeria; Kaldor, Uzi; Eliav, Ephraim. "Fully relativistic ab initio studies of superheavy elements" (PDF). www.kernchemie.uni-mainz.de. Johannes Gutenberg University Mainz. Archived from the original (PDF) on January 15, 2018. Retrieved January 15, 2018.
- Gäggeler, H. W. (2007). "Gas Phase Chemistry of Superheavy Elements" (PDF). Paul Scherrer Institute. pp. 26–28. Archived from the original (PDF) on February 20, 2012.
- Moody, Ken (November 30, 2013). "Synthesis of Superheavy Elements". In Schädel, Matthias; Shaughnessy, Dawn (eds.). The Chemistry of Superheavy Elements (2nd ed.). Springer Science & Business Media. pp. 24–8. ISBN 9783642374661.
- Zaitsevskii, A.; van Wüllen, C.; Rusakov, A.; Titov, A. (September 2007). "Relativistic DFT and ab initio calculations on the seventh-row superheavy elements: E113 – E114" (PDF). jinr.ru. Retrieved February 17, 2018.
- Paul Scherrer Institute (2015). "Annual Report 2015: Laboratory of Radiochemistry and Environmental Chemistry" (PDF). Paul Scherrer Institute. p. 3.
{{cite web}}
: CS1 maint: uses authors parameter (link)
Bibliography
- Audi, G.; Kondev, F. G.; Wang, M.; Huang, W. J.; Naimi, S. (2017). "The NUBASE2016 evaluation of nuclear properties". Chinese Physics C. 41 (3). 030001. Bibcode:2017ChPhC..41c0001A. doi:10.1088/1674-1137/41/3/030001.
- Beiser, A. (2003). Concepts of modern physics (6th ed.). McGraw-Hill. ISBN 978-0-07-244848-1. OCLC 48965418.
- Hoffman, D. C.; Ghiorso, A.; Seaborg, G. T. (2000). The Transuranium People: The Inside Story. World Scientific. ISBN 978-1-78-326244-1.
- Kragh, H. (2018). From Transuranic to Superheavy Elements: A Story of Dispute and Creation. Springer. ISBN 978-3-319-75813-8.
- Zagrebaev, Valeriy; Karpov, Alexander; Greiner, Walter (2013). "Future of superheavy element research: Which nuclei could be synthesized within the next few years?" (PDF). 11th International Conference on Nucleus-Nucleus Collisions (NN2012). Journal of Physics: Conference Series. Vol. 420. IOP Publishing. doi:10.1088/1742-6596/420/1/012001. Retrieved August 20, 2013.
External links


- Copernicium at The Periodic Table of Videos (University of Nottingham)