Ganymede (moon)
Ganymede, a satellite of Jupiter (Jupiter III), is the largest and most massive of the Solar System's moons. The ninth-largest object (including the Sun) of the Solar System, it is the largest without a substantial atmosphere (albeit not the most massive one, this is Mercury). It has a diameter of 5,268 km (3,273 mi), making it 26 percent larger than the planet Mercury by volume, although it is only 45 percent as massive.[16] Possessing a metallic core, it has the lowest moment of inertia factor of any solid body in the Solar System and is the only moon known to have a magnetic field. Outward from Jupiter, it is the seventh satellite and the third of the Galilean moons, the first group of objects discovered orbiting another planet.[17] Ganymede orbits Jupiter in roughly seven days and is in a 1:2:4 orbital resonance with the moons Europa and Io, respectively.
![]() Ganymede photographed by Juno in 2021 | |||||||||||||
Discovery[1][2] | |||||||||||||
---|---|---|---|---|---|---|---|---|---|---|---|---|---|
Discovered by | Galileo Galilei | ||||||||||||
Discovery date | January 7, 1610 | ||||||||||||
Designations | |||||||||||||
Pronunciation | /ˈɡænəˌmiːd/[3] GAN-ə-MEED | ||||||||||||
Named after | Γανυμήδης, Ganymēdēs | ||||||||||||
Alternative names | Jupiter III | ||||||||||||
Adjectives | Ganymedian,[4] Ganymedean[5][6] (/ˌɡænəˈmiːdi.ən/) | ||||||||||||
Orbital characteristics | |||||||||||||
Periapsis | 1069200 km[lower-alpha 1] | ||||||||||||
Apoapsis | 1071600 km[lower-alpha 2] | ||||||||||||
1070400 km[7] | |||||||||||||
Eccentricity | 0.0013[7] | ||||||||||||
7.15455296 d[7] | |||||||||||||
Average orbital speed | 10.880 km/s | ||||||||||||
Inclination | 2.214° (to the ecliptic) 0.20° (to Jupiter's equator)[7] | ||||||||||||
Satellite of | Jupiter | ||||||||||||
Group | Galilean moon | ||||||||||||
Physical characteristics | |||||||||||||
Mean radius | 2634.1±0.3 km (0.413 Earths)[8] | ||||||||||||
8.72×107 km2 (0.171 Earths)[lower-alpha 3] | |||||||||||||
Volume | 7.66×1010 km3 (0.0704 Earths)[lower-alpha 4] | ||||||||||||
Mass | 1.4819×1023 kg (0.025 Earths)[8] | ||||||||||||
Mean density | 1.936 g/cm3 (0.351 Earths)[8] | ||||||||||||
Surface gravity | 1.428 m/s2 (0.146 g)[lower-alpha 5] | ||||||||||||
Moment of inertia factor | 0.3115±0.0028[9] | ||||||||||||
2.741 km/s[lower-alpha 6] | |||||||||||||
Synodic rotation period | synchronous | ||||||||||||
0–0.33°[10] | |||||||||||||
Albedo | 0.43±0.02[11] | ||||||||||||
| |||||||||||||
4.61 (opposition)[11] 4.38 (in 1951)[14] | |||||||||||||
1.2 to 1.8 arcseconds | |||||||||||||
Atmosphere | |||||||||||||
Surface pressure | 0.2–1.2 μPa (1.97×10−12–1.18×10−11 atm)[15] | ||||||||||||
Composition by volume | mostly oxygen[15] | ||||||||||||
Ganymede is composed of approximately equal amounts of silicate rock and water. It is a fully differentiated body with an iron-rich, liquid core, and an internal ocean that may contain more water than all of Earth's oceans combined.[18][19][20][21] Its surface is composed of two main types of terrain. Dark regions, saturated with impact craters and dated to four billion years ago, cover about a third of it. Lighter regions, crosscut by extensive grooves and ridges and only slightly less ancient, cover the remainder. The cause of the light terrain's disrupted geology is not fully known, but was likely the result of tectonic activity due to tidal heating.[8]
Ganymede's magnetic field is probably created by convection within its liquid iron core, also created by Jupiter's tidal forces.[22] The meager magnetic field is buried within Jupiter's far larger magnetic field and would show only as a local perturbation of the field lines. Ganymede has a thin oxygen atmosphere that includes O, O2, and possibly O3 (ozone).[15] Atomic hydrogen is a minor atmospheric constituent. Whether Ganymede has an ionosphere associated with its atmosphere is unresolved.[23]
Ganymede's discovery is credited to Galileo Galilei, the first to observe it, on January 7, 1610.[1][lower-alpha 7] Its name was soon suggested by astronomer Simon Marius, after the mythological Ganymede, a Trojan prince desired by Zeus (the Greek counterpart of Jupiter), who carried him off to be the cupbearer of the gods.[25] Beginning with Pioneer 10, several spacecraft have explored Ganymede.[26] The Voyager probes, Voyager 1 and Voyager 2, refined measurements of its size, while Galileo discovered its underground ocean and magnetic field. The next planned mission to the Jovian system is the European Space Agency's Jupiter Icy Moon Explorer (JUICE), due to launch in 2023.[27] After flybys of all three icy Galilean moons, it is planned to enter orbit around Ganymede.[28]
History
Chinese astronomical records report that in 365 BC, Gan De detected what might have been a moon of Jupiter, probably Ganymede, with the naked eye.[29][30] However, Gan De reported the color of the companion as reddish, which is puzzling since the moons are too faint for their color to be perceived with the naked eye.[31] Shi Shen and Gan De together made fairly accurate observations of the five major planets.[32][33]
On January 7, 1610, Galileo Galilei used a telescope to observe what he thought were three stars near Jupiter, including what turned out to be Ganymede, Callisto, and one body that turned out to be the combined light from Io and Europa; the next night he noticed that they had moved. On January 13, he saw all four at once for the first time, but had seen each of the moons before this date at least once. By January 15, Galileo came to the conclusion that the stars were actually bodies orbiting Jupiter.[1][2][lower-alpha 7]
Name
Galileo claimed the right to name the moons he had discovered. He considered "Cosmian Stars" and settled on "Medicean Stars", in honor of Cosimo II de' Medici.[25]
The French astronomer Nicolas-Claude Fabri de Peiresc suggested individual names from the Medici family for the moons, but his proposal was not taken up.[25] Simon Marius, who had originally claimed to have found the Galilean satellites,[34] tried to name the moons the "Saturn of Jupiter", the "Jupiter of Jupiter" (this was Ganymede), the "Venus of Jupiter", and the "Mercury of Jupiter", another nomenclature that never caught on. From a suggestion by Johannes Kepler, Marius suggested a different naming system based on Greek mythology:[25]
... Quin etiam impensius amavit Ganymedem puerum formosum, Trois Regis filium, adeo etiam assumptâ aquilæ figurâ, illum humeris impositum, in cœlum transportavit, prout fabulantur poetæ... à me vocatur... Tertius ob luminis Majestatem Ganymedes... [Io,] Europa, Ganimedes puer, atque Calisto, lascivo nimium perplacuere Jovi.
... Then there was Ganymede, the handsome son of King Tros, whom Jupiter, having taken the form of an eagle, transported to heaven on his back, as poets fabulously tell ... The Third [moon] is called Ganymede by me on account of its majesty of light ... Io, Europa, the boy Ganymede, and Callisto greatly pleased lustful Zeus.[35][36]
This name and those of the other Galilean satellites fell into disfavor for a considerable time, and were not in common use until the mid-20th century. In much of the earlier astronomical literature, Ganymede is referred to instead by its Roman numeral designation, Jupiter III (a system introduced by Galileo), in other words "the third satellite of Jupiter". Following the discovery of moons of Saturn, a naming system based on that of Kepler and Marius was used for Jupiter's moons.[25] Ganymede is the only Galilean moon of Jupiter named after a male figure—like Io, Europa, and Callisto, he was a lover of Zeus.
The Galilean satellites retain the Italian spellings of their names. In the cases of Io, Europa and Callisto, these are identical to the Latin, but the Latin form of Ganymede is Ganymedes. In English, the final 'e' is silent, perhaps under the influence of French, unlike later names taken from Latin and Greek.
Orbit and rotation
Ganymede orbits Jupiter at a distance of 1,070,400 kilometres (665,100 mi), third among the Galilean satellites,[17] and completes a revolution every seven days and three hours. Like most known moons, Ganymede is tidally locked, with one side always facing toward the planet, hence its day is also seven days and three hours.[37] Its orbit is very slightly eccentric and inclined to the Jovian equator, with the eccentricity and inclination changing quasi-periodically due to solar and planetary gravitational perturbations on a timescale of centuries. The ranges of change are 0.0009–0.0022 and 0.05–0.32°, respectively.[38] These orbital variations cause the axial tilt (the angle between rotational and orbital axes) to vary between 0 and 0.33°.[10]
Ganymede participates in orbital resonances with Europa and Io: for every orbit of Ganymede, Europa orbits twice and Io orbits four times.[38][39] Conjunctions (alignment on the same side of Jupiter) between Io and Europa occur when Io is at periapsis and Europa at apoapsis. Conjunctions between Europa and Ganymede occur when Europa is at periapsis.[38] The longitudes of the Io–Europa and Europa–Ganymede conjunctions change with the same rate, making triple conjunctions impossible. Such a complicated resonance is called the Laplace resonance.[40] The current Laplace resonance is unable to pump the orbital eccentricity of Ganymede to a higher value.[40] The value of about 0.0013 is probably a remnant from a previous epoch, when such pumping was possible.[39] The Ganymedian orbital eccentricity is somewhat puzzling; if it is not pumped now it should have decayed long ago due to the tidal dissipation in the interior of Ganymede.[40] This means that the last episode of the eccentricity excitation happened only several hundred million years ago.[40] Because Ganymede's orbital eccentricity is relatively low—on average 0.0015[39]—tidal heating is negligible now.[40] However, in the past Ganymede may have passed through one or more Laplace-like resonances[lower-alpha 8] that were able to pump the orbital eccentricity to a value as high as 0.01–0.02.[8][40] This probably caused a significant tidal heating of the interior of Ganymede; the formation of the grooved terrain may be a result of one or more heating episodes.[8][40]
There are two hypotheses for the origin of the Laplace resonance among Io, Europa, and Ganymede: that it is primordial and has existed from the beginning of the Solar System;[41] or that it developed after the formation of the Solar System. A possible sequence of events for the latter scenario is as follows: Io raised tides on Jupiter, causing Io's orbit to expand (due to conservation of momentum) until it encountered the 2:1 resonance with Europa; after that the expansion continued, but some of the angular moment was transferred to Europa as the resonance caused its orbit to expand as well; the process continued until Europa encountered the 2:1 resonance with Ganymede.[40] Eventually the drift rates of conjunctions between all three moons were synchronized and locked in the Laplace resonance.[40]
Physical characteristics
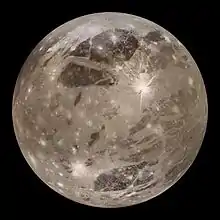
.png.webp)
Size
With a diameter of about 5,270 kilometres (3,270 mi) and a mass of 1.48×1020 tonnes (1.48×1023 kg; 3.26×1023 lb), Ganymede is the largest and most massive moon in the Solar System.[42] It is slightly more massive than the second most massive moon, Saturn's satellite Titan, and is more than twice as massive as the Earth's Moon. It is larger than the planet Mercury, which has a diameter of 4,880 kilometres (3,030 mi), but is only 45 percent of Mercury's mass. Ganymede is the ninth-largest object in the solar system, but the tenth-most massive.
Composition
The average density of Ganymede, 1.936 g/cm3 (a bit greater than Callisto's), suggests a composition of about equal parts rocky material and mostly water ices.[8] Some of the water is liquid, forming an underground ocean.[43] The mass fraction of ices is between 46 and 50 percent, which is slightly lower than that in Callisto.[44] Some additional volatile ices such as ammonia may also be present.[44][45] The exact composition of Ganymede's rock is not known, but is probably close to the composition of L/LL type ordinary chondrites,[44] which are characterized by less total iron, less metallic iron and more iron oxide than H chondrites. The weight ratio of iron to silicon ranges between 1.05 and 1.27 in Ganymede, whereas the solar ratio is around 1.8.[44]
Surface features
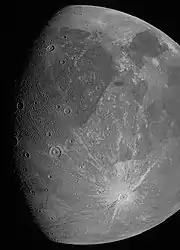
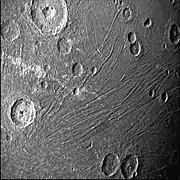

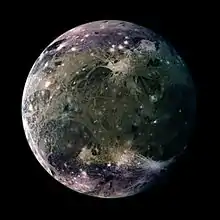
Ganymede's surface has an albedo of about 43 percent.[47] Water ice seems to be ubiquitous on its surface, with a mass fraction of 50–90 percent,[8] significantly more than in Ganymede as a whole. Near-infrared spectroscopy has revealed the presence of strong water ice absorption bands at wavelengths of 1.04, 1.25, 1.5, 2.0 and 3.0 μm.[47] The grooved terrain is brighter and has a more icy composition than the dark terrain.[48] The analysis of high-resolution, near-infrared and UV spectra obtained by the Galileo spacecraft and from Earth observations has revealed various non-water materials: carbon dioxide, sulfur dioxide and, possibly, cyanogen, hydrogen sulfate and various organic compounds.[8][49] Galileo results have also shown magnesium sulfate (MgSO4) and, possibly, sodium sulfate (Na2SO4) on Ganymede's surface.[37][50] These salts may originate from the subsurface ocean.[50]
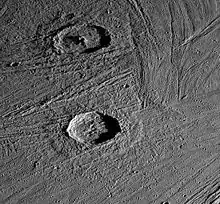
The Ganymedian surface albedo is very asymmetric; the leading hemisphere[lower-alpha 9] is brighter than the trailing one.[47] This is similar to Europa, but the reverse for Callisto.[47] The trailing hemisphere of Ganymede appears to be enriched in sulfur dioxide.[51][52] The distribution of carbon dioxide does not demonstrate any hemispheric asymmetry, although it is not observed near the poles.[49][53] Impact craters on Ganymede (except one) do not show any enrichment in carbon dioxide, which also distinguishes it from Callisto. Ganymede's carbon dioxide gas was probably depleted in the past.[53]
Ganymede's surface is a mix of two types of terrain: very old, highly cratered, dark regions and somewhat younger (but still ancient), lighter regions marked with an extensive array of grooves and ridges. The dark terrain, which comprises about one-third of the surface,[54] contains clays and organic materials that could indicate the composition of the impactors from which Jovian satellites accreted.[55]
The heating mechanism required for the formation of the grooved terrain on Ganymede is an unsolved problem in the planetary sciences. The modern view is that the grooved terrain is mainly tectonic in nature.[8] Cryovolcanism is thought to have played only a minor role, if any.[8] The forces that caused the strong stresses in the Ganymedian ice lithosphere necessary to initiate the tectonic activity may be connected to the tidal heating events in the past, possibly caused when the satellite passed through unstable orbital resonances.[8][56] The tidal flexing of the ice may have heated the interior and strained the lithosphere, leading to the development of cracks and horst and graben faulting, which erased the old, dark terrain on 70 percent of the surface.[8][57] The formation of the grooved terrain may also be connected with the early core formation and subsequent tidal heating of Ganymede's interior, which may have caused a slight expansion of Ganymede by one to six percent due to phase transitions in ice and thermal expansion.[8] During subsequent evolution deep, hot water plumes may have risen from the core to the surface, leading to the tectonic deformation of the lithosphere.[58] Radiogenic heating within the satellite is the most relevant current heat source, contributing, for instance, to ocean depth. Research models have found that if the orbital eccentricity were an order of magnitude greater than currently (as it may have been in the past), tidal heating would be a more substantial heat source than radiogenic heating.[59]
Cratering is seen on both types of terrain, but is especially extensive on the dark terrain: it appears to be saturated with impact craters and has evolved largely through impact events.[8] The brighter, grooved terrain contains many fewer impact features, which have been only of a minor importance to its tectonic evolution.[8] The density of cratering indicates an age of 4 billion years for the dark terrain, similar to the highlands of the Moon, and a somewhat younger age for the grooved terrain (but how much younger is uncertain).[60] Ganymede may have experienced a period of heavy cratering 3.5 to 4 billion years ago similar to that of the Moon.[60] If true, the vast majority of impacts happened in that epoch, whereas the cratering rate has been much smaller since.[61] Craters both overlay and are crosscut by the groove systems, indicating that some of the grooves are quite ancient. Relatively young craters with rays of ejecta are also visible.[61][62] Ganymedian craters are flatter than those on the Moon and Mercury. This is probably due to the relatively weak nature of Ganymede's icy crust, which can (or could) flow and thereby soften the relief. Ancient craters whose relief has disappeared leave only a "ghost" of a crater known as a palimpsest.[61]
One significant feature on Ganymede is a dark plain named Galileo Regio, which contains a series of concentric grooves, or furrows, likely created during a period of geologic activity.[63]
Ganymede also has polar caps, likely composed of water frost. The frost extends to 40° latitude.[37] These polar caps were first seen by the Voyager spacecraft. Theories on the formation of the caps include the migration of water to higher latitudes and bombardment of the ice by plasma. Data from Galileo suggests the latter is correct.[64] The presence of a magnetic field on Ganymede results in more intense charged particle bombardment of its surface in the unprotected polar regions; sputtering then leads to redistribution of water molecules, with frost migrating to locally colder areas within the polar terrain.[64]
A crater named Anat provides the reference point for measuring longitude on Ganymede. By definition, Anat is at 128° longitude.[65] The 0° longitude directly faces Jupiter, and unless stated otherwise longitude increases toward the west.[66]
Internal structure
Ganymede appears to be fully differentiated, with an internal structure consisting of an iron-sulfide–iron core, a silicate mantle and outer layers of water ice and liquid water.[8][67] [68] The precise thicknesses of the different layers in the interior of Ganymede depend on the assumed composition of silicates (fraction of olivine and pyroxene) and amount of sulfur in the core.[44][67][69] Ganymede has the lowest moment of inertia factor, 0.31,[8] among the solid Solar System bodies. This is a consequence of its substantial water content and fully differentiated interior.
Subsurface oceans
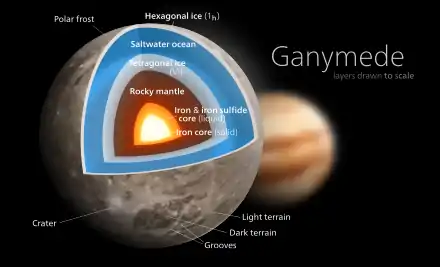
In the 1970s, NASA scientists first suspected that Ganymede has a thick ocean between two layers of ice, one on the surface and one beneath a liquid ocean and atop the rocky mantle.[8][19][67][70][71] In the 1990s, NASA's Galileo mission flew by Ganymede, and found indications of such a subsurface ocean.[43] An analysis published in 2014, taking into account the realistic thermodynamics for water and effects of salt, suggests that Ganymede might have a stack of several ocean layers separated by different phases of ice, with the lowest liquid layer adjacent to the rocky mantle.[19][20][21][72] Water–rock contact may be an important factor in the origin of life.[19] The analysis also notes that the extreme depths involved (~800 km to the rocky "seafloor") mean that temperatures at the bottom of a convective (adiabatic) ocean can be up to 40 K higher than those at the ice–water interface.
In March 2015, scientists reported that measurements with the Hubble Space Telescope of how the aurorae moved confirmed that Ganymede has a subsurface ocean.[43] A large salt-water ocean affects Ganymede's magnetic field, and consequently, its aurora.[18][72][73][74] The evidence suggests that Ganymede's oceans might be the largest in the entire Solar System.[75]
There is some speculation on the potential habitability of Ganymede's ocean.[71][76]
Core
The existence of a liquid, iron–nickel-rich core[68] provides a natural explanation for the intrinsic magnetic field of Ganymede detected by Galileo spacecraft.[77] The convection in the liquid iron, which has high electrical conductivity, is the most reasonable model of magnetic field generation.[22] The density of the core is 5.5–6 g/cm3 and the silicate mantle is 3.4–3.6 g/cm3.[44][67][69][77] The radius of this core may be up to 500 km.[77] The temperature in the core of Ganymede is probably 1500–1700 K and pressure up to 10 GPa (99,000 atm).[67][77]
Atmosphere and ionosphere
In 1972, a team of Indian, British and American astronomers working in Java (Indonesia) and Kavalur (India) claimed that they had detected a thin atmosphere during an occultation, when it and Jupiter passed in front of a star.[78] They estimated that the surface pressure was around 0.1 Pa (1 microbar).[78] However, in 1979, Voyager 1 observed an occultation of the star κ Centauri during its flyby of Jupiter, with differing results.[79] The occultation measurements were conducted in the far-ultraviolet spectrum at wavelengths shorter than 200 nm, which were much more sensitive to the presence of gases than the 1972 measurements made in the visible spectrum. No atmosphere was revealed by the Voyager data. The upper limit on the surface particle number density was found to be 1.5×109 cm−3, which corresponds to a surface pressure of less than 2.5 µPa (25 picobar).[79] The latter value is almost five orders of magnitude less than the 1972 estimate.[79]
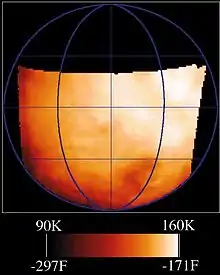
Despite the Voyager data, evidence for a tenuous oxygen atmosphere (exosphere) on Ganymede, very similar to the one found on Europa, was found by the Hubble Space Telescope (HST) in 1995.[15][80] HST actually observed airglow of atomic oxygen in the far-ultraviolet at the wavelengths 130.4 nm and 135.6 nm. Such an airglow is excited when molecular oxygen is dissociated by electron impacts,[15] which is evidence of a significant neutral atmosphere composed predominantly of O2 molecules. The surface number density probably lies in the (1.2–7)×108 cm−3 range, corresponding to the surface pressure of 0.2–1.2 µPa.[15][lower-alpha 10] These values are in agreement with the Voyager's upper limit set in 1981. The oxygen is not evidence of life; it is thought to be produced when water ice on Ganymede's surface is split into hydrogen and oxygen by radiation, with the hydrogen then being more rapidly lost due to its low atomic mass.[80] The airglow observed over Ganymede is not spatially homogeneous like that over Europa. HST observed two bright spots located in the northern and southern hemispheres, near ± 50° latitude, which is exactly the boundary between the open and closed field lines of the Ganymedian magnetosphere (see below).[81] The bright spots are probably polar auroras, caused by plasma precipitation along the open field lines.[82]
The existence of a neutral atmosphere implies that an ionosphere should exist, because oxygen molecules are ionized by the impacts of the energetic electrons coming from the magnetosphere[83] and by solar EUV radiation.[23] However, the nature of the Ganymedian ionosphere is as controversial as the nature of the atmosphere. Some Galileo measurements found an elevated electron density near Ganymede, suggesting an ionosphere, whereas others failed to detect anything.[23] The electron density near the surface is estimated by different sources to lie in the range 400–2,500 cm−3.[23] As of 2008, the parameters of the ionosphere of Ganymede are not well constrained.
Additional evidence of the oxygen atmosphere comes from spectral detection of gases trapped in the ice at the surface of Ganymede. The detection of ozone (O3) bands was announced in 1996.[84] In 1997 spectroscopic analysis revealed the dimer (or diatomic) absorption features of molecular oxygen. Such an absorption can arise only if the oxygen is in a dense phase. The best candidate is molecular oxygen trapped in ice. The depth of the dimer absorption bands depends on latitude and longitude, rather than on surface albedo—they tend to decrease with increasing latitude on Ganymede, whereas O3 shows an opposite trend.[85] Laboratory work has found that O2 would not cluster or bubble but dissolve in ice at Ganymede's relatively warm surface temperature of 100 K (−173.15 °C).[86]
A search for sodium in the atmosphere, just after such a finding on Europa, turned up nothing in 1997. Sodium is at least 13 times less abundant around Ganymede than around Europa, possibly because of a relative deficiency at the surface or because the magnetosphere fends off energetic particles.[87] Another minor constituent of the Ganymedian atmosphere is atomic hydrogen. Hydrogen atoms were observed as far as 3,000 km from Ganymede's surface. Their density on the surface is about 1.5×104 cm−3.[88]
In 2021 water vapour was detected in the atmosphere of Ganymede.[89]
Magnetosphere
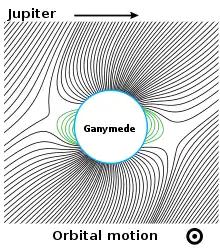
The Galileo craft made six close flybys of Ganymede from 1995 to 2000 (G1, G2, G7, G8, G28 and G29)[22] and discovered that Ganymede has a permanent (intrinsic) magnetic moment independent of the Jovian magnetic field.[90]The value of the moment is about 1.3 × 1013 T·m3,[22] which is three times larger than the magnetic moment of Mercury. The magnetic dipole is tilted with respect to the rotational axis of Ganymede by 176°, which means that it is directed against the Jovian magnetic moment.[22] Its north pole lies below the orbital plane. The dipole magnetic field created by this permanent moment has a strength of 719 ± 2 nT at Ganymede's equator,[22] which should be compared with the Jovian magnetic field at the distance of Ganymede—about 120 nT.[90] The equatorial field of Ganymede is directed against the Jovian field, meaning reconnection is possible. The intrinsic field strength at the poles is two times that at the equator—1440 nT.[22]
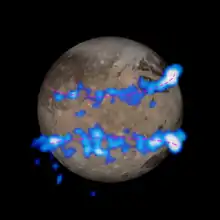
The permanent magnetic moment carves a part of space around Ganymede, creating a tiny magnetosphere embedded inside that of Jupiter; it is the only moon in the Solar System known to possess the feature.[90] Its diameter is 4–5 Ganymede radii.[91] The Ganymedian magnetosphere has a region of closed field lines located below 30° latitude, where charged particles (electrons and ions) are trapped, creating a kind of radiation belt.[91] The main ion species in the magnetosphere is single ionized oxygen—O+[23]—which fits well with Ganymede's tenuous oxygen atmosphere. In the polar cap regions, at latitudes higher than 30°, magnetic field lines are open, connecting Ganymede with Jupiter's ionosphere.[91] In these areas, the energetic (tens and hundreds of kiloelectronvolt) electrons and ions have been detected,[83] which may cause the auroras observed around the Ganymedian poles.[81] In addition, heavy ions precipitate continuously on Ganymede's polar surface, sputtering and darkening the ice.[83]
The interaction between the Ganymedian magnetosphere and Jovian plasma is in many respects similar to that of the solar wind and Earth's magnetosphere.[91][92]The plasma co-rotating with Jupiter impinges on the trailing side of the Ganymedian magnetosphere much like the solar wind impinges on the Earth's magnetosphere. The main difference is the speed of plasma flow—supersonic in the case of Earth and subsonic in the case of Ganymede. Because of the subsonic flow, there is no bow shock off the trailing hemisphere of Ganymede.[92]
In addition to the intrinsic magnetic moment, Ganymede has an induced dipole magnetic field.[22] Its existence is connected with the variation of the Jovian magnetic field near Ganymede. The induced moment is directed radially to or from Jupiter following the direction of the varying part of the planetary magnetic field. The induced magnetic moment is an order of magnitude weaker than the intrinsic one. The field strength of the induced field at the magnetic equator is about 60 nT—half of that of the ambient Jovian field.[22] The induced magnetic field of Ganymede is similar to those of Callisto and Europa, indicating that Ganymede also has a subsurface water ocean with a high electrical conductivity.[22]
Given that Ganymede is completely differentiated and has a metallic core,[8][77] its intrinsic magnetic field is probably generated in a similar fashion to the Earth's: as a result of conducting material moving in the interior.[22][77] The magnetic field detected around Ganymede is likely to be caused by compositional convection in the core,[77] if the magnetic field is the product of dynamo action, or magnetoconvection.[22][93]
Despite the presence of an iron core, Ganymede's magnetosphere remains enigmatic, particularly given that similar bodies lack the feature.[8] Some research has suggested that, given its relatively small size, the core ought to have sufficiently cooled to the point where fluid motions, hence a magnetic field would not be sustained. One explanation is that the same orbital resonances proposed to have disrupted the surface also allowed the magnetic field to persist: with Ganymede's eccentricity pumped and tidal heating of the mantle increased during such resonances, reducing heat flow from the core, leaving it fluid and convective.[57] Another explanation is a remnant magnetization of silicate rocks in the mantle, which is possible if the satellite had a more significant dynamo-generated field in the past.[8]
Radiation environment
The radiation level at the surface of Ganymede is considerably lower than at Europa, being 50–80 mSv (5–8 rem) per day, an amount that would cause severe illness or death in human beings exposed for two months.[94]
Origin and evolution
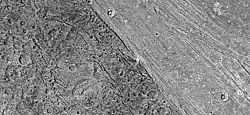
Ganymede probably formed by an accretion in Jupiter's subnebula, a disk of gas and dust surrounding Jupiter after its formation.[95] The accretion of Ganymede probably took about 10,000 years,[96] much shorter than the 100,000 years estimated for Callisto. The Jovian subnebula may have been relatively "gas-starved" when the Galilean satellites formed; this would have allowed for the lengthy accretion times required for Callisto.[95] In contrast Ganymede formed closer to Jupiter, where the subnebula was denser, which explains its shorter formation timescale.[96] This relatively fast formation prevented the escape of accretional heat, which may have led to ice melt and differentiation: the separation of the rocks and ice. The rocks settled to the center, forming the core.[68] In this respect, Ganymede is different from Callisto, which apparently failed to melt and differentiate early due to loss of the accretional heat during its slower formation.[97] This hypothesis explains why the two Jovian moons look so dissimilar, despite their similar mass and composition.[70][97] Alternative theories explain Ganymede's greater internal heating on the basis of tidal flexing[98] or more intense pummeling by impactors during the Late Heavy Bombardment.[99][100][101][102] In the latter case, modeling suggests that differentiation would become a runaway process at Ganymede but not Callisto.[101][102]
After formation, Ganymede's core largely retained the heat accumulated during accretion and differentiation, only slowly releasing it to the ice mantle.[97] The mantle, in turn, transported it to the surface by convection.[70] The decay of radioactive elements within rocks further heated the core, causing increased differentiation: an inner, iron–iron-sulfide core and a silicate mantle formed.[77][97] With this, Ganymede became a fully differentiated body.[68] By comparison, the radioactive heating of undifferentiated Callisto caused convection in its icy interior, which effectively cooled it and prevented large-scale melting of ice and rapid differentiation.[103] The convective motions in Callisto have caused only a partial separation of rock and ice.[103] Today, Ganymede continues to cool slowly.[77] The heat being released from its core and silicate mantle enables the subsurface ocean to exist,[45] whereas the slow cooling of the liquid Fe–FeS core causes convection and supports magnetic field generation.[77] The current heat flux out of Ganymede is probably higher than that out of Callisto.[97]
Exploration
Several spacecraft have performed close flybys of Ganymede: two Pioneer and two Voyager spacecraft made a single flyby each between 1973 and 1979; the Galileo spacecraft made six passes between 1996 and 2000; and the Juno spacecraft performed two flybys in 2019 and 2021.[104] No spacecraft has yet orbited Ganymede, but there have been multiple proposals to do so, including the JUICE mission which is currently under construction (as of 2021).
Completed flybys
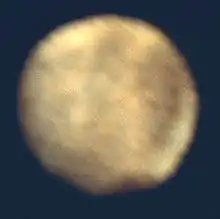
The first spacecraft to approach close to Ganymede was Pioneer 10, which performed a flyby in 1973 as it passed through the Jupiter system at high speed. Pioneer 11 made a similar flyby in 1974.[26] Data sent back by the two spacecraft was used to determine the moon's physical characteristics[105] and provided images of the surface with up to 400 km (250 mi) resolution.[106] Pioneer 10's closest approach was 446,250 km, about 85 times Ganymede's diameter.[107]
Voyager 1 and Voyager 2 both studied Ganymede when passing through the Jupiter system in 1979. Data from those flybys were used to refine the size of Ganymede, revealing it was larger than Saturn's moon Titan, which was previously thought to have been bigger.[108] Images from the Voyagers provided the first views of the moon's grooved surface terrain.[109]
The Pioneer and Voyager flybys were all at large distances and high speeds, as they flew on unbound trajectories through the Jupiter system. Better data can be obtained from a spacecraft which is orbiting Jupiter, as it can encounter Ganymede at a lower speed and adjust the orbit for a closer approach. In 1995, the Galileo spacecraft entered orbit around Jupiter and between 1996 and 2000 made six close flybys of Ganymede.[37] These flybys were denoted G1, G2, G7, G8, G28 and G29.[22] During the closest flyby (G2), Galileo passed just 264 km from the surface of Ganymede (five percent of the moon's diameter),[22] which remains the closest approach by any spacecraft. During the G1 flyby in 1996, Galileo instruments detected Ganymede's magnetic field.[110] Data from the Galileo flybys was used to discover the sub-surface ocean, which was announced in 2001.[22][37] High spatial resolution spectra of Ganymede taken by Galileo were used to identify several non-ice compounds on the surface.[49]
The New Horizons spacecraft also observed Ganymede, but from a much larger distance as it passed through the Jupiter system in 2007 (en route to Pluto). The data were used to perform topographic and compositional mapping of Ganymede.[111][112]
Like Galileo, the Juno spacecraft orbited around Jupiter. On 2019 December 25, Juno performed a distant flyby of Ganymede during its 24th orbit of Jupiter, at a range of 97,680 to 109,439 kilometers (60,696 to 68,002 mi). This flyby provided images of the moon's polar regions.[113][114] In June 2021, Juno performed a second flyby, at a closer distance of 1,038 kilometers (645 mi).[104][115] This encounter was designed to provide a gravity assist to reduce Juno's orbital period from 53 days to 43 days. Additional images of the surface were collected.[104]
Future missions
The Jupiter Icy Moons Explorer (JUICE) will be the first to enter orbit around Ganymede itself. As of 2021, JUICE is under construction, with launch planned for August 2023.[27][116] It is intended to perform its first flyby of Ganymede in 2031, then enter orbit of the moon in 2032. When the spacecraft consumes its propellant, JUICE is planned to be deorbited and impact Ganymede in February 2034.[117]
Proposed missions
Several other missions have been proposed to flyby or orbit Ganymede, but were either not selected for funding or cancelled before launch.
The Jupiter Icy Moons Orbiter would have studied Ganymede in greater detail.[118] However, the mission was canceled in 2005.[119] Another old proposal was called The Grandeur of Ganymede.[55]
A Ganymede orbiter based on the Juno probe was proposed in 2010 for the Planetary Science Decadal Survey.[120] The mission was not supported, with the Decadal Survey preferring the Europa Clipper mission instead.[121]
The Europa Jupiter System Mission (EJSM) had a proposed launch date in 2020, and was a joint NASA and ESA proposal for exploration of many of Jupiter's moons including Ganymede. In February 2009 it was announced that ESA and NASA had given this mission priority ahead of the Titan Saturn System Mission.[122] EJSM was to consist of the NASA-led Jupiter Europa Orbiter, the ESA-led Jupiter Ganymede Orbiter, and possibly a JAXA-led Jupiter Magnetospheric Orbiter. The NASA and JAXA components were later cancelled, and ESA's appeared likely to be cancelled too,[123] but in 2012 ESA announced it would go ahead alone. The European part of the mission became the Jupiter Icy Moon Explorer (JUICE) (see above).[124]
The Russian Space Research Institute proposed a Ganymede lander (GL) astrobiology mission called Laplace-P,[125] possibly in partnership with JUICE.[125][126] If selected, it would be launched in 2023.[125]
Gallery
- Hubble Space Telescope image of Ganymede taken in 1996.[127]
- Infrared image of Ganymede taken during the Juno flyby in July 2021. Image Credits: A. Mura -Juno/JIRAM - ASI/INAF/JPL-Caltech/SwRI [114]
See also
- Cold trap (astronomy)
- Jupiter's moons in fiction
- List of craters on Ganymede
- List of geological features on Ganymede
- List of natural satellites
- Lunar and Planetary Institute
Notes
- Periapsis is derived from the semimajor axis (a) and eccentricity (e): .
- Apoapsis is derived from the semimajor axis (a) and eccentricity (e): .
- Surface area derived from the radius (r): .
- Volume derived from the radius (r): .
- Surface gravity derived from the mass (m), the gravitational constant (G) and the radius (r): .
- Escape velocity derived from the mass (m), the gravitational constant (G) and the radius (r): .
- It is probable that the German astronomer Simon Marius discovered it independently the same year.[24]
- A Laplace-like resonance is similar to the current Laplace resonance among the Galilean moons with the only difference being that longitudes of the Io–Europa and Europa–Ganymede conjunctions change with rates whose ratio is a non-unity rational number. If the ratio is unity, then the resonance is the Laplace resonance.
- The leading hemisphere is the hemisphere facing the direction of orbital motion; the trailing hemisphere faces the reverse direction.
- The surface number density and pressure were calculated from the column densities reported in Hall, et al. 1998, assuming a scale height of 20 km and temperature 120 K.
References
- Galilei, Galileo; translated by Edward Carlos (March 1610). Barker, Peter (ed.). "Sidereus Nuncius" (PDF). University of Oklahoma History of Science. Archived from the original (PDF) on December 20, 2005. Retrieved January 13, 2010.
- "In Depth | Ganymede". NASA Solar System Exploration. Retrieved June 16, 2021.
- "Ganymede". Oxford English Dictionary (Online ed.). Oxford University Press. (Subscription or participating institution membership required.)
"Ganymede". Merriam-Webster Dictionary. - Quinn Passey & E. M. Shoemaker (1982) "Craters on Ganymede and Callisto", in David Morrison, ed., Satellites of Jupiter, vol. 3, International Astronomical Union, pp. 385–386, 411.
- Journal of Geophysical Research, v. 95 (1990).
- E. M. Shoemaker et al. (1982) "Geology of Ganymede", in David Morrison, ed., Satellites of Jupiter, vol. 3, International Astronomical Union, pp. 464, 482, 496.
- "Planetary Satellite Mean Orbital Parameters". Jet Propulsion Laboratory, California Institute of Technology.
- Showman, Adam P.; Malhotra, Renu (October 1, 1999). "The Galilean Satellites" (PDF). Science. 286 (5437): 77–84. doi:10.1126/science.286.5437.77. PMID 10506564.
- Schubert, G.; Anderson, J. D.; Spohn, T.; McKinnon, W. B. (2004). "Interior composition, structure and dynamics of the Galilean satellites". In Bagenal, F.; Dowling, T. E.; McKinnon, W. B. (eds.). Jupiter: the planet, satellites, and magnetosphere. New York: Cambridge University Press. pp. 281–306. ISBN 978-0521035453. OCLC 54081598.
- Bills, Bruce G. (2005). "Free and forced obliquities of the Galilean satellites of Jupiter". Icarus. 175 (1): 233–247. Bibcode:2005Icar..175..233B. doi:10.1016/j.icarus.2004.10.028.
- Yeomans, Donald K. (July 13, 2006). "Planetary Satellite Physical Parameters". JPL Solar System Dynamics. Retrieved November 5, 2007.
- Delitsky, Mona L.; Lane, Arthur L. (1998). "Ice chemistry of Galilean satellites" (PDF). J. Geophys. Res. 103 (E13): 31, 391–31, 403. Bibcode:1998JGR...10331391D. doi:10.1029/1998JE900020. Archived from the original (PDF) on October 3, 2006.
- Orton, G. S.; Spencer, G. R.; et al. (1996). "Galileo Photopolarimeter-radiometer observations of Jupiter and the Galilean Satellites". Science. 274 (5286): 389–391. Bibcode:1996Sci...274..389O. doi:10.1126/science.274.5286.389. S2CID 128624870.
- Yeomans; Chamberlin. "Horizon Online Ephemeris System for Ganymede (Major Body 503)". California Institute of Technology, Jet Propulsion Laboratory. Retrieved April 14, 2010. (4.38 on 1951-Oct-03).
- Hall, D. T.; Feldman, P. D.; et al. (1998). "The Far-Ultraviolet Oxygen Airglow of Europa and Ganymede". The Astrophysical Journal. 499 (1): 475–481. Bibcode:1998ApJ...499..475H. doi:10.1086/305604.
- "Ganymede Fact Sheet". www2.jpl.nasa.gov. Archived from the original on January 5, 1997. Retrieved January 14, 2010.
- "Jupiter's Moons". The Planetary Society. Archived from the original on December 31, 2007.
- Staff (March 12, 2015). "NASA's Hubble Observations Suggest Underground Ocean on Jupiter's Largest Moon". NASA News. Retrieved March 15, 2015.
- Clavin, Whitney (May 1, 2014). "Ganymede May Harbor 'Club Sandwich' of Oceans and Ice". NASA. Jet Propulsion Laboratory. Retrieved May 1, 2014.
- Vance, Steve; Bouffard, Mathieu; Choukroun, Mathieu; Sotina, Christophe (April 12, 2014). "Ganymede's internal structure including thermodynamics of magnesium sulfate oceans in contact with ice". Planetary and Space Science. 96: 62–70. Bibcode:2014P&SS...96...62V. doi:10.1016/j.pss.2014.03.011.
- Staff (May 1, 2014). "Video (00:51) - Jupiter's 'Club Sandwich' Moon". NASA. Archived from the original on November 14, 2021. Retrieved May 2, 2014.
- Kivelson, M.G.; Khurana, K.K.; et al. (2002). "The Permanent and Inductive Magnetic Moments of Ganymede" (PDF). Icarus. 157 (2): 507–522. Bibcode:2002Icar..157..507K. doi:10.1006/icar.2002.6834. hdl:2060/20020044825. S2CID 7482644.
- Eviatar, Aharon; Vasyliunas, Vytenis M.; et al. (2001). "The ionosphere of Ganymede" (ps). Planet. Space Sci. 49 (3–4): 327–336. Bibcode:2001P&SS...49..327E. doi:10.1016/S0032-0633(00)00154-9.
- "Ganymede (satellite of Jupiter)". Encyclopædia Britannica. Retrieved November 19, 2019.
- "Satellites of Jupiter". The Galileo Project. Retrieved November 24, 2007.
- "Pioneer 11". Solar System Exploration. Archived from the original on September 2, 2011. Retrieved January 6, 2008.
- "ESA Science & Technology – JUICE". ESA. November 8, 2021. Retrieved November 10, 2021.
- Amos, Jonathan (May 2, 2012). "Esa selects 1bn-euro Juice probe to Jupiter". BBC News. Retrieved May 2, 2012.
- Chamberlain, V. D. (1981). "Astronomical content of American Plains Indian winter counts". Bulletin of the Astronomical Society. 13: 793. Bibcode:1981BAAS...13..793C.
- Brecher, K. (1981). "Ancient Astronomy in Modern China". Bulletin of the Astronomical Society. 13: 793. Bibcode:1981BAAS...13..793B.
- Yi-Long, Huang (1997). "Gan De". In Helaine Selin (ed.). Encyclopaedia of the history of science, technology, and medicine in non-western cultures. Springer. p. 342. ISBN 978-0-7923-4066-9.
- Yinke Deng (March 3, 2011). Ancient Chinese Inventions. Cambridge University Press. p. 68. ISBN 978-0-521-18692-6.
- Xi, Ze-zong (1981). "The Discovery of Jupiter's Satellite Made by Gan De 2000 Years Before Galileo". Acta Astrophysica Sinica. 1 (2): 87. Bibcode:1981AcApS...1...85X. Retrieved March 22, 2017.
- "Discovery". Cascadia Community College. Archived from the original on September 20, 2006. Retrieved November 24, 2007.
- Marius, Simon (1614). Mundus Iovialis: anno MDCIX detectus ope perspicilli Belgici, hoc est, quatuor Jovialium planetarum, cum theoria, tum tabulæ. Nuremberg: Sumptibus & Typis Iohannis Lauri. p. B2, recto and verso (images 35 and 36), with erratum on last page (image 78). Retrieved June 30, 2020.
- "The Discovery of the Galilean Satellites". Views of the Solar System. Space Research Institute, Russian Academy of Sciences. Archived from the original on November 18, 2007. Retrieved November 24, 2007.
- Miller, Ron; Hartmann, William K. (May 2005). The Grand Tour: A Traveler's Guide to the Solar System (3rd ed.). Thailand: Workman Publishing. pp. 108–114. ISBN 978-0-7611-3547-0.
- Musotto, Susanna; Varadi, Ferenc; Moore, William; Schubert, Gerald (2002). "Numerical Simulations of the Orbits of the Galilean Satellites". Icarus. 159 (2): 500–504. Bibcode:2002Icar..159..500M. doi:10.1006/icar.2002.6939.
- Phillips, Cynthia (October 3, 2002). "High Tide on Europa". SPACE.com. Archived from the original on October 17, 2002.
- Showman, Adam P.; Malhotra, Renu (1997). "Tidal Evolution into the Laplace Resonance and the Resurfacing of Ganymede" (PDF). Icarus. 127 (1): 93–111. Bibcode:1997Icar..127...93S. doi:10.1006/icar.1996.5669.
- Peale, S.J.; Lee, Man Hoi (2002). "A Primordial Origin of the Laplace Relation Among the Galilean Satellites". Science. 298 (5593): 593–597. arXiv:astro-ph/0210589. Bibcode:2002Sci...298..593P. doi:10.1126/science.1076557. PMID 12386333. S2CID 18590436.
- "Ganymede". NASA Solar System Exploration. Retrieved June 15, 2021.
- Chang, Kenneth (March 12, 2015). "Suddenly, It Seems, Water Is Everywhere in Solar System". New York Times. Retrieved March 12, 2015.
- Kuskov, O.L.; Kronrod, V.A. (2005). "Internal structure of Europa and Callisto". Icarus. 177 (2): 550–569. Bibcode:2005Icar..177..550K. doi:10.1016/j.icarus.2005.04.014.
- Spohn, T.; Schubert, G. (2003). "Oceans in the icy Galilean satellites of Jupiter?" (PDF). Icarus. 161 (2): 456–467. Bibcode:2003Icar..161..456S. doi:10.1016/S0019-1035(02)00048-9. Archived from the original (PDF) on February 27, 2008.
- "Galileo has successful flyby of Ganymede during eclipse". Spaceflight Now. Retrieved January 19, 2008.
- Calvin, Wendy M.; Clark, Roger N.; Brown, Robert H.; Spencer, John R. (1995). "Spectra of the ice Galilean satellites from 0.2 to 5 µm: A compilation, new observations, and a recent summary". J. Geophys. Res. 100 (E9): 19, 041–19, 048. Bibcode:1995JGR...10019041C. doi:10.1029/94JE03349.
- "Ganymede: the Giant Moon". Wayne RESA. Archived from the original on December 2, 2007. Retrieved December 31, 2007.
- McCord, T.B.; Hansen, G.V.; et al. (1998). "Non-water-ice constituents in the surface material of the icy Galilelean satellites from Galileo near-infrared mapping spectrometer investigation". J. Geophys. Res. 103 (E4): 8, 603–8, 626. Bibcode:1998JGR...103.8603M. doi:10.1029/98JE00788.
- McCord, Thomas B.; Hansen, Gary B.; Hibbitts, Charles A. (2001). "Hydrated Salt Minerals on Ganymede's Surface: Evidence of an Ocean Below". Science. 292 (5521): 1523–1525. Bibcode:2001Sci...292.1523M. doi:10.1126/science.1059916. PMID 11375486. S2CID 40346198.
- Domingue, Deborah; Lane, Arthur; Moth, Pimol (1996). "Evidence from IUE for Spatial and Temporal Variations in the Surface Composition of the Icy Galilean Satellites". Bulletin of the American Astronomical Society. 28: 1070. Bibcode:1996DPS....28.0404D.
- Domingue, Deborah L.; Lane, Arthur L.; Beyer, Ross A. (1998). "IEU's detection of tenuous SO2 frost on Ganymede and its rapid time variability". Geophys. Res. Lett. 25 (16): 3, 117–3, 120. Bibcode:1998GeoRL..25.3117D. doi:10.1029/98GL02386. S2CID 128823420.
- Hibbitts, C.A.; Pappalardo, R.; Hansen, G.V.; McCord, T.B. (2003). "Carbon dioxide on Ganymede". J. Geophys. Res. 108 (E5): 5, 036. Bibcode:2003JGRE..108.5036H. doi:10.1029/2002JE001956.
- Patterson, Wesley; Head, James W.; et al. (2007). "A Global Geologic Map of Ganymede" (PDF). Lunar and Planetary Science. XXXVIII: 1098.
- Pappalardo, R.T.; Khurana, K.K.; Moore, W.B. (2001). "The Grandeur of Ganymede: Suggested Goals for an Orbiter Mission" (PDF). Lunar and Planetary Science. XXXII: 4062. Bibcode:2001iaop.work...62P.
- Showman, Adam P.; Stevenson, David J.; Malhotra, Renu (1997). "Coupled Orbital and Thermal Evolution of Ganymede" (PDF). Icarus. 129 (2): 367–383. Bibcode:1997Icar..129..367S. doi:10.1006/icar.1997.5778.
- Bland; Showman, A.P.; Tobie, G. (March 2007). "Ganymede's orbital and thermal evolution and its effect on magnetic field generation" (PDF). Lunar and Planetary Society Conference. 38 (1338): 2020. Bibcode:2007LPI....38.2020B.
- Barr, A.C.; Pappalardo, R. T.; Pappalardo, Stevenson (2001). "Rise of Deep Melt into Ganymede's Ocean and Implications for Astrobiology" (PDF). Lunar and Planetary Science Conference. 32: 1781. Bibcode:2001LPI....32.1781B.
- Huffmann, H.; et al. (2004). "Internal Structure and Tidal Heating of Ganymede" (PDF). Geophysical Research Abstracts. 6.
- Zahnle, K.; Dones, L. (1998). "Cratering Rates on the Galilean Satellites" (PDF). Icarus. 136 (2): 202–222. Bibcode:1998Icar..136..202Z. doi:10.1006/icar.1998.6015. PMID 11878353. Archived from the original (PDF) on February 27, 2008.
- "Ganymede". nineplanets.org. October 31, 1997. Retrieved February 27, 2008.
- "Ganymede". Lunar and Planetary Institute. 1997.
- Casacchia, R.; Strom, R.G. (1984). "Geologic evolution of Galileo Regio". Journal of Geophysical Research. 89: B419–B428. Bibcode:1984LPSC...14..419C. doi:10.1029/JB089iS02p0B419.
- Khurana, Krishan K.; Pappalardo, Robert T.; Murphy, Nate; Denk, Tilmann (2007). "The origin of Ganymede's polar caps". Icarus. 191 (1): 193–202. Bibcode:2007Icar..191..193K. doi:10.1016/j.icarus.2007.04.022.
- "USGS Astrogeology: Rotation and pole position for planetary satellites (IAU WGCCRE)". Archived from the original on October 24, 2011. Retrieved August 28, 2017.
- "Planetary Names: Target Coordinate Systems". planetarynames.wr.usgs.gov. International Astronomical Union. Archived from the original on May 27, 2016. Retrieved May 21, 2016.
- Sohl, F.; Spohn, T; Breuer, D.; Nagel, K. (2002). "Implications from Galileo Observations on the Interior Structure and Chemistry of the Galilean Satellites". Icarus. 157 (1): 104–119. Bibcode:2002Icar..157..104S. doi:10.1006/icar.2002.6828.
- Bhatia, G.K.; Sahijpal, S. (2017). "Thermal evolution of trans-Neptunian objects, icy satellites, and minor icy planets in the early solar system". Meteoritics & Planetary Science. 52 (12): 2470–2490. Bibcode:2017M&PS...52.2470B. doi:10.1111/maps.12952. S2CID 133957919.
- Kuskov, O.L.; Kronrod, V.A.; Zhidikova, A.P. (2005). Internal Structure of Icy Satellites of Jupiter (PDF). Geophysical Research Abstracts. Vol. 7. p. 01892. Bibcode:2010aogs...19..365K. doi:10.1142/9789812838162_0028. ISBN 9789812838162.
- Freeman, J. (2006). "Non-Newtonian stagnant lid convection and the thermal evolution of Ganymede and Callisto" (PDF). Planetary and Space Science. 54 (1): 2–14. Bibcode:2006P&SS...54....2F. doi:10.1016/j.pss.2005.10.003. Archived from the original (PDF) on August 24, 2007.
- "Underground ocean on Jupiter's largest moon". EarthSky. March 15, 2015. Retrieved August 14, 2015.
- "Hubble observations suggest underground ocean on Jupiter's largest moon Ganymede". NASA. PhysOrg. March 12, 2015. Retrieved March 13, 2015.
- "Underground ocean on Jupiter's largest moon, Ganymede".
- Saur, Joachim; Duling, Stefan; Roth, Lorenz; Jia, Xianzhe; Strobel, Darrell F.; Feldman, Paul D.; Christensen, Ulrich R.; Retherford, Kurt D.; McGrath, Melissa A.; Musacchio, Fabrizio; Wennmacher, Alexandre; Neubauer, Fritz M.; Simon, Sven; Hartkorn, Oliver (2015). "The Search for a Subsurface Ocean in Ganymede with Hubble Space Telescope Observations of its Auroral Ovals". Journal of Geophysical Research: Space Physics. 120 (3): 1715–1737. Bibcode:2015JGRA..120.1715S. doi:10.1002/2014JA020778. hdl:2027.42/111157.
- Wenz, John (October 4, 2017). "Overlooked Ocean Worlds Fill the Outer Solar System". Scientific American.
- Griffin, Andrew (March 13, 2015). "Ganymede: oceans on Jupiter's moon could have been home to alien life". The Independent. Archived from the original on March 13, 2015. Retrieved February 19, 2018.
- Hauck, Steven A.; Aurnou, Jonathan M.; Dombard, Andrew J. (2006). "Sulfur's impact on core evolution and magnetic field generation on Ganymede". J. Geophys. Res. 111 (E9): E09008. Bibcode:2006JGRE..111.9008H. doi:10.1029/2005JE002557.
- Carlson, R.W.; Bhattacharyya, J. C.; et al. (1973). "Atmosphere of Ganymede from its occultation of SAO 186800 on 7 June 1972" (PDF). Science. 182 (4107): 53–5. Bibcode:1973Sci...182...53C. doi:10.1126/science.182.4107.53. PMID 17829812. S2CID 33370778.
- Broadfoot, A.L.; Sandel, B.R.; et al. (1981). "Overview of the Voyager Ultraviolet Spectrometry Results through Jupiter Encounter" (PDF). Journal of Geophysical Research. 86 (A10): 8259–8284. Bibcode:1981JGR....86.8259B. doi:10.1029/JA086iA10p08259.
- "Hubble Finds Thin Oxygen Atmosphere on Ganymede". Jet Propulsion Laboratory. NASA. October 23, 1996. Archived from the original on May 4, 2009. Retrieved February 17, 2017.
- Feldman, Paul D.; McGrath, Melissa A.; et al. (2000). "HST/STIS Ultraviolet Imaging of Polar Aurora on Ganymede". The Astrophysical Journal. 535 (2): 1085–1090. arXiv:astro-ph/0003486. Bibcode:2000ApJ...535.1085F. doi:10.1086/308889. S2CID 15558538.
- Johnson, R.E. (1997). "Polar "Caps" on Ganymede and Io Revisited". Icarus. 128 (2): 469–471. Bibcode:1997Icar..128..469J. doi:10.1006/icar.1997.5746.
- Paranicas, C.; Paterson, W. R.; et al. (1999). "Energetic particles observations near Ganymede". J. Geophys. Res. 104 (A8): 17, 459–17, 469. Bibcode:1999JGR...10417459P. doi:10.1029/1999JA900199.
- Noll, Keith S.; Johnson, Robert E.; et al. (July 1996). "Detection of Ozone on Ganymede". Science. 273 (5273): 341–343. Bibcode:1996Sci...273..341N. doi:10.1126/science.273.5273.341. PMID 8662517. S2CID 32074586.
- Calvin, Wendy M.; Spencer, John R. (December 1997). "Latitudinal Distribution of O2 on Ganymede: Observations with the Hubble Space Telescope". Icarus. 130 (2): 505–516. Bibcode:1997Icar..130..505C. doi:10.1006/icar.1997.5842.
- Vidal, R. A.; et al. (1997). "Oxygen on Ganymede: Laboratory Studies". Science. 276 (5320): 1839–1842. Bibcode:1997Sci...276.1839V. doi:10.1126/science.276.5320.1839. PMID 9188525. S2CID 27378519.
- Brown, Michael E. (1997). "A Search for a Sodium Atmosphere around Ganymede". Icarus. 126 (1): 236–238. Bibcode:1997Icar..126..236B. CiteSeerX 10.1.1.24.7010. doi:10.1006/icar.1996.5675.
- Barth, C.A.; Hord, C.W.; et al. (1997). "Galileo ultraviolet spectrometer observations of atomic hydrogen in the atmosphere of Ganymede". Geophys. Res. Lett. 24 (17): 2147–2150. Bibcode:1997GeoRL..24.2147B. doi:10.1029/97GL01927. S2CID 123038216.
- Water vapor detected on huge Jupiter moon Ganymede for 1st time, Space.com
- Kivelson, M.G.; Khurana, K.K.; et al. (1997). "The magnetic field and magnetosphere of Ganymede" (PDF). Geophys. Res. Lett. 24 (17): 2155–2158. Bibcode:1997GeoRL..24.2155K. doi:10.1029/97GL02201.
- Kivelson, M.G.; Warnecke, J.; et al. (1998). "Ganymede's magnetosphere: magnetometer overview" (PDF). J. Geophys. Res. 103 (E9): 19, 963–19, 972. Bibcode:1998JGR...10319963K. doi:10.1029/98JE00227.
- Volwerk, M.; Kivelson, M.G.; Khurana, K.K.; McPherron, R.L. (1999). "Probing Ganymede's magnetosphere with field line resonances" (PDF). J. Geophys. Res. 104 (A7): 14, 729–14, 738. Bibcode:1999JGR...10414729V. doi:10.1029/1999JA900161.
- Hauck, Steven A.; Dombard, A. J.; Solomon, S. C.; Aurnou, J. M. (2002). "Internal structure and mechanism of core convection on Ganymede" (PDF). Lunar and Planetary Science. XXXIII: 1380. Bibcode:2002LPI....33.1380H.
- Podzolko, M.V.; Getselev, I.V. (March 8, 2013). "Radiation Conditions of a Mission to Jupiterʼs Moon Ganymede". International Colloquium and Workshop "Ganymede Lander: Scientific Goals and Experiments. IKI, Moscow, Russia: Moscow State University. Retrieved January 6, 2020.
- Canup, Robin M.; Ward, William R. (2002). "Formation of the Galilean Satellites: Conditions of Accretion" (PDF). The Astronomical Journal. 124 (6): 3404–3423. Bibcode:2002AJ....124.3404C. doi:10.1086/344684. S2CID 47631608.
- Mosqueira, Ignacio; Estrada, Paul R (2003). "Formation of the regular satellites of giant planets in an extended gaseous nebula I: subnebula model and accretion of satellites". Icarus. 163 (1): 198–231. Bibcode:2003Icar..163..198M. doi:10.1016/S0019-1035(03)00076-9.
- McKinnon, William B. (2006). "On convection in ice I shells of outer Solar System bodies, with detailed application to Callisto". Icarus. 183 (2): 435–450. Bibcode:2006Icar..183..435M. doi:10.1016/j.icarus.2006.03.004.
- Showman, A. P.; Malhotra, R. (March 1997). "Tidal evolution into the Laplace resonance and the resurfacing of Ganymede". Icarus. 127 (1): 93–111. Bibcode:1997Icar..127...93S. doi:10.1006/icar.1996.5669. S2CID 55790129.
- Baldwin, E. (January 25, 2010). "Comet impacts explain Ganymede-Callisto dichotomy". Astronomy Now. Retrieved March 1, 2010.
- "Researchers offer explanation for the differences between Ganymede and Callisto moons". Phys.Org. January 24, 2010. Retrieved February 3, 2017.
- Barr, A. C.; Canup, R. M. (March 2010). Origin of the Ganymede/Callisto dichotomy by impacts during an outer solar system late heavy bombardment (PDF). 41st Lunar and Planetary Science Conference (2010). Houston. Retrieved March 1, 2010.
- Barr, A. C.; Canup, R. M. (January 24, 2010). "Origin of the Ganymede–Callisto dichotomy by impacts during the late heavy bombardment" (PDF). Nature Geoscience. 3 (March 2010): 164–167. Bibcode:2010NatGe...3..164B. CiteSeerX 10.1.1.827.982. doi:10.1038/NGEO746.
- Nagel, K.A; Breuer, D.; Spohn, T. (2004). "A model for the interior structure, evolution, and differentiation of Callisto". Icarus. 169 (2): 402–412. Bibcode:2004Icar..169..402N. doi:10.1016/j.icarus.2003.12.019.
- Chang, Kenneth (June 8, 2021). "NASA Just Visited the Solar System's Biggest Moon - The Juno spacecraft completed a close flyby of Ganymede, Jupiter's biggest moon, as it transitions into a new phase of its mission". The New York Times. Archived from the original on December 28, 2021. Retrieved June 10, 2021.
- "Exploration of Ganymede". Terraformers Society of Canada. Archived from the original on March 19, 2007. Retrieved January 6, 2008.
- "Chapter 6: Results at the New Frontiers". SP-349/396 Pioneer Odyssey. NASA. August 1974.
- "Pioneer 10 Full Mission Timeline". D Muller. Archived from the original on July 23, 2011. Retrieved May 25, 2011.
- "Voyager 1 and 2". ThinkQuest. Archived from the original on December 26, 2007. Retrieved January 6, 2008.
- "The Voyager Planetary Mission". Views of the Solar System. Archived from the original on February 3, 2008. Retrieved January 6, 2008.
- "New Discoveries From Galileo". Jet Propulsion Laboratory. Archived from the original on January 5, 1997. Retrieved January 6, 2008.
- "Pluto-Bound New Horizons Spacecraft Gets A Boost From Jupiter". Space Daily. Retrieved January 6, 2008.
- Grundy, W.M.; Buratti, B.J.; et al. (2007). "New Horizons Mapping of Europa and Ganymede". Science. 318 (5848): 234–237. Bibcode:2007Sci...318..234G. doi:10.1126/science.1147623. PMID 17932288. S2CID 21071030.
- "Ganymede". Southwest Research Institute. January 9, 2020. Retrieved January 10, 2020.
- Inaf, Ufficio stampa (August 6, 2021). "Gli occhi di Jiram sull'equatore di Ganimede". MEDIA INAF (in Italian). Retrieved December 8, 2021.
- "Nasa spacecraft captures first closeups of Jupiter's largest moon in decades". The Guardian. Associated Press. June 8, 2021. Retrieved June 9, 2021.
- Witasse, O.; Altobelli, N.; Andres, R.; Atzei, A.; Boutonnet, A.; Budnik, F.; Dietz, A.; Erd, C.; Evill, R.; Lorente, R.; Munoz, C.; Pinzan, G.; Scharmberg, C.; Suarez, A.; Tanco, I.; Torelli, F.; Torn, B.; Vallat, C.; JUICE Science Working Team (July 2021). JUICE (Jupiter Icy Moon Explorer): Plans for the cruise phase. Europlanet Science Congress (EPSC) 2021. doi:10.5194/epsc2021-358. Retrieved August 28, 2021.
- Elizabeth Howell (February 14, 2017). "JUICE: Exploring Jupiter's Moons". Space.com. Retrieved February 3, 2022.
- "Jupiter Icy Moons Orbiter (JIMO)". The Internet Encyclopedia of Science. Retrieved January 6, 2008.
- Peplow, M. (February 8, 2005). "NASA budget kills Hubble telescope". Nature. doi:10.1038/news050207-4. Retrieved December 24, 2011.
- "Planetary Science Decadal Survey Mission & Technology Studies". Space Studies Board. Archived from the original on April 28, 2014. Retrieved November 12, 2012. "Ganymede Orbiter" (PDF).
- National Research Council (March 7, 2011). Vision and Voyages for Planetary Science in the Decade 2013-2022. Washington DC, USA: The National Academies Press. doi:10.17226/13117. ISBN 978-0-309-22464-2.
The committee identified a number of additional large missions that are of high scientific value but are not recommended for the decade 2013-2022 for a variety of reasons. In alphabetical order, these missions are as follows: Ganymede Orbiter [...]
- Rincon, Paul (February 20, 2009). "Jupiter in space agencies' sights". BBC News. Retrieved February 20, 2009.
- "Cosmic Vision 2015–2025 Proposals". ESA. July 21, 2007. Retrieved February 20, 2009.
- "ESA – Selection of the L1 mission" (PDF). ESA. April 17, 2012. Retrieved April 15, 2014.
- "International Colloquium and Workshop – "Ganymede Lander: scientific goals and experiments"". Russia Space Research Institute (IKI). Roscosmos. November 2012. Retrieved November 20, 2012.
- Amos, Jonathan (November 20, 2012). "Russia and Europe joint Mars bid agreement approved". BBC News.
- "Hubble Finds First Evidence of Water Vapour at Jupiter's Moon Ganymede". Retrieved August 3, 2021.
Further reading
- Dougherty; Grasset (2011). Jupiter Icy Moon Explorer (PDF).
- "Jupiter's Great Red Spot and Ganymede's shadow". www.spacetelescope.org. ESA/Hubble. Retrieved October 31, 2014.
External links

- Ganymede page at NASA's Solar System Exploration site
- Ganymede page at The Nine Planets
- Ganymede page at Views of the Solar System
- Ganymede Crater Database from the Lunar and Planetary Institute
- Images of Ganymede at JPL's Planetary Photojournal
- Movie of Ganymede's rotation from the National Oceanic and Atmospheric Administration
- Ganymede map from Scientific American article
- Ganymede map with feature names from Planetary Photojournal
- Ganymede nomenclature and Ganymede map with feature names from the USGS planetary nomenclature page
- Paul Schenk's 3D images and flyover videos of Ganymede and other outer solar system satellites
- Ganymede Orbiter Concept
- Global Geologic Map of Ganymede (USGS)
- Google Ganymede 3D, interactive map of the moon
- Video (animation; 4:00): Flyby of Ganymede and Jupiter (NASA; July 15, 2021).