Adenylyl cyclase
Adenylate cyclase (EC 4.6.1.1, also commonly known as adenyl cyclase and adenylyl cyclase, abbreviated AC) is an enzyme with systematic name ATP diphosphate-lyase (cyclizing; 3′,5′-cyclic-AMP-forming). It catalyzes the following reaction:
- ATP = 3′,5′-cyclic AMP + diphosphate
Adenylate cyclase | |||||||||
---|---|---|---|---|---|---|---|---|---|
![]() Adenylate cyclase (calmodulin sensitive) trimer, Bacillus anthracis Epinephrine binds its receptor, that associates with a heterotrimeric G protein. The G protein associates with adenylyl cyclase, which converts ATP to cAMP, spreading the signal.[1] | |||||||||
Identifiers | |||||||||
EC no. | 4.6.1.1 | ||||||||
CAS no. | 9012-42-4 | ||||||||
Databases | |||||||||
IntEnz | IntEnz view | ||||||||
BRENDA | BRENDA entry | ||||||||
ExPASy | NiceZyme view | ||||||||
KEGG | KEGG entry | ||||||||
MetaCyc | metabolic pathway | ||||||||
PRIAM | profile | ||||||||
PDB structures | RCSB PDB PDBe PDBsum | ||||||||
Gene Ontology | AmiGO / QuickGO | ||||||||
|
It has key regulatory roles in essentially all cells.[2] It is the most polyphyletic known enzyme: six distinct classes have been described, all catalyzing the same reaction but representing unrelated gene families with no known sequence or structural homology.[3] The best known class of adenylyl cyclases is class III or AC-III (Roman numerals are used for classes). AC-III occurs widely in eukaryotes and has important roles in many human tissues.[4]
All classes of adenylyl cyclase catalyse the conversion of adenosine triphosphate (ATP) to 3',5'-cyclic AMP (cAMP) and pyrophosphate.[4] Magnesium ions are generally required and appear to be closely involved in the enzymatic mechanism. The cAMP produced by AC then serves as a regulatory signal via specific cAMP-binding proteins, either transcription factors, enzymes (e.g., cAMP-dependent kinases), or ion transporters.
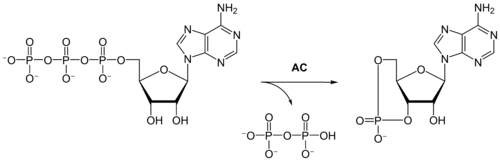
Classes
Class I
Adenylate cyclase, class-I | |||||||||
---|---|---|---|---|---|---|---|---|---|
Identifiers | |||||||||
Symbol | Adenylate_cycl | ||||||||
Pfam | PF01295 | ||||||||
InterPro | IPR000274 | ||||||||
PROSITE | PDOC00837 | ||||||||
|
The first class of adenylyl cyclases occur in many bacteria including E. coli (as CyaA P00936 [unrelated to the Class II enzyme]).[4] This was the first class of AC to be characterized. It was observed that E. coli deprived of glucose produce cAMP that serves as an internal signal to activate expression of genes for importing and metabolizing other sugars. cAMP exerts this effect by binding the transcription factor CRP, also known as CAP. Class I AC's are large cytosolic enzymes (~100 kDa) with a large regulatory domain (~50 kDa) that indirectly senses glucose levels. As of 2012, no crystal structure is available for class I AC.
Some indirect structural information is available for this class. It is known that the N-terminal half is the catalytic portion, and that it requires two Mg2+ ions. S103, S113, D114, D116 and W118 are the five absolutely essential residues. The class I catalytic domain (Pfam PF12633) belongs to the same superfamily (Pfam CL0260) as the palm domain of DNA polymerase beta (Pfam PF18765). Aligning its sequence onto the structure onto a related archaeal CCA tRNA nucleotidyltransferase (PDB: 1R89) allows for assignment of the residues to specific functions: γ-phosphate binding, structural stabilization, DxD motif for metal ion binding, and finally ribose binding.[5]
Class II
These adenylyl cyclases are toxins secreted by pathogenic bacteria such as Bacillus anthracis, Bordetella pertussis, Pseudomonas aeruginosa, and Vibrio vulnificus during infections.[6] These bacteria also secrete proteins that enable the AC-II to enter host cells, where the exogenous AC activity undermines normal cellular processes. The genes for Class II ACs are known as cyaA, one of which is anthrax toxin. Several crystal structures are known for AC-II enzymes.[7][8][9]
Class III
Adenylyl cyclase class-3/guanylyl cyclase | |||||||||
---|---|---|---|---|---|---|---|---|---|
Identifiers | |||||||||
Symbol | Guanylate_cyc | ||||||||
Pfam | PF00211 | ||||||||
Pfam clan | CL0276 | ||||||||
InterPro | IPR001054 | ||||||||
SMART | SM00044 | ||||||||
PROSITE | PS50125 | ||||||||
SCOP2 | 1tl7 / SCOPe / SUPFAM | ||||||||
TCDB | 8.A.85 | ||||||||
OPM superfamily | 546 | ||||||||
OPM protein | 6r3q | ||||||||
|
These adenylyl cyclases are the most familiar based on extensive study due to their important roles in human health. They are also found in some bacteria, notably Mycobacterium tuberculosis where they appear to have a key role in pathogenesis. Most AC-III's are integral membrane proteins involved in transducing extracellular signals into intracellular responses. A Nobel Prize was awarded to Earl Sutherland in 1971 for discovering the key role of AC-III in human liver, where adrenaline indirectly stimulates AC to mobilize stored energy in the "fight or flight" response. The effect of adrenaline is via a G protein signaling cascade, which transmits chemical signals from outside the cell across the membrane to the inside of the cell (cytoplasm). The outside signal (in this case, adrenaline) binds to a receptor, which transmits a signal to the G protein, which transmits a signal to adenylyl cyclase, which transmits a signal by converting adenosine triphosphate to cyclic adenosine monophosphate (cAMP). cAMP is known as a second messenger.[10]
Cyclic AMP is an important molecule in eukaryotic signal transduction, a so-called second messenger. Adenylyl cyclases are often activated or inhibited by G proteins, which are coupled to membrane receptors and thus can respond to hormonal or other stimuli.[11] Following activation of adenylyl cyclase, the resulting cAMP acts as a second messenger by interacting with and regulating other proteins such as protein kinase A and cyclic nucleotide-gated ion channels.[11]
Photoactivated adenylyl cyclase (PAC) was discovered in Euglena gracilis and can be expressed in other organisms through genetic manipulation. Shining blue light on a cell containing PAC activates it and abruptly increases the rate of conversion of ATP to cAMP. This is a useful technique for researchers in neuroscience because it allows them to quickly increase the intracellular cAMP levels in particular neurons, and to study the effect of that increase in neural activity on the behavior of the organism.[12] A green-light activated rhodopsin adenylyl cyclase (CaRhAC) has recently been engineered by modifying the nucleotide binding pocket of rhodopsin guanylyl cyclase.
Structure
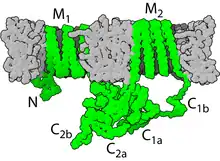
Most class III adenylyl cyclases are transmembrane proteins with 12 transmembrane segments. The protein is organized with 6 transmembrane segments, then the C1 cytoplasmic domain, then another 6 membrane segments, and then a second cytoplasmic domain called C2. The important parts for function are the N-terminus and the C1 and C2 regions. The C1a and C2a subdomains are homologous and form an intramolecular 'dimer' that forms the active site. In Mycobacterium tuberculosis and many other bacterial cases, the AC-III polypeptide is only half as long, comprising one 6-transmembrane domain followed by a cytoplasmic domain, but two of these form a functional homodimer that resembles the mammalian architecture with two active sites. In non-animal class III ACs, the catalytic cytoplasmic domain is seen associated with other (not necessarily transmembrane) domains.[13]
Class III adenylyl cyclase domains can be further divided into four subfamilies, termed class IIIa through IIId. Animal membrane-bound ACs belong to class IIIa.[13]: 1087
Mechanism
The reaction happens with two metal cofactors (Mg or Mn) coordinated to the two aspartate residues on C1. They perform a nucleophilic attack of the 3'-OH group of the ribose on the α-phosphoryl group of ATP. The two lysine and aspartate residues on C2 selects ATP over GTP for the substrate, so that the enzyme is not a guanylyl cyclase. A pair of arginine and asparagine residues on C2 stabilizes the transition state. In many proteins, these residues are nevertheless mutated while retaining the adenylyl cyclase activity.[13]
Types
There are ten known isoforms of adenylyl cyclases in mammals:
These are also sometimes called simply AC1, AC2, etc., and, somewhat confusingly, sometimes Roman numerals are used for these isoforms that all belong to the overall AC class III. They differ mainly in how they are regulated, and are differentially expressed in various tissues throughout mammalian development.
Regulation
Adenylyl cyclase is regulated by G proteins, which can be found in the monomeric form or the heterotrimeric form, consisting of three subunits.[2][3][4] Adenylyl cyclase activity is controlled by heterotrimeric G proteins.[2][3][4] The inactive or inhibitory form exists when the complex consists of alpha, beta, and gamma subunits, with GDP bound to the alpha subunit.[2][4] In order to become active, a ligand must bind to the receptor and cause a conformational change.[2] This conformational change causes the alpha subunit to dissociate from the complex and become bound to GTP.[2] This G-alpha-GTP complex then binds to adenylyl cyclase and causes activation and the release of cAMP.[2] Since a good signal requires the help of enzymes, which turn on and off signals quickly, there must also be a mechanism in which adenylyl cyclase deactivates and inhibits cAMP.[2] The deactivation of the active G-alpha-GTP complex is accomplished rapidly by GTP hydrolysis due to the reaction being catalyzed by the intrinsic enzymatic activity of GTPase located in the alpha subunit.[2] It is also regulated by forskolin,[11] as well as other isoform-specific effectors:
- Isoforms I, III, and VIII are also stimulated by Ca2+/calmodulin.[11]
- Isoforms V and VI are inhibited by Ca2+ in a calmodulin-independent manner.[11]
- Isoforms II, IV and IX are stimulated by alpha subunit of the G protein.[11]
- Isoforms I, V and VI are most clearly inhibited by Gi, while other isoforms show less dual regulation by the inhibitory G protein.[11]
- Soluble AC (sAC) is not a transmembrane form and is not regulated by G proteins or forskolin, instead acts as a bicarbonate/pH sensor. It is anchored at various locations within the cell and, with phosphodiesterases, forms local cAMP signalling domains.[14]
In neurons, calcium-sensitive adenylyl cyclases are located next to calcium ion channels for faster reaction to Ca2+ influx; they are suspected of playing an important role in learning processes. This is supported by the fact that adenylyl cyclases are coincidence detectors, meaning that they are activated only by several different signals occurring together.[15] In peripheral cells and tissues adenylyl cyclases appear to form molecular complexes with specific receptors and other signaling proteins in an isoform-specific manner.
Function
Individual transmembrane adenylyl cyclase isoforms have been linked to numerous physiological functions.[16] Soluble adenylyl cyclase (sAC, AC10) has a critical role in sperm motility.[17] Adenylyl cyclase has been implicated in memory formation, functioning as a coincidence detector.[11][15][18][19][20]
Class IV
Adenylyl cyclase CyaB | |
---|---|
Identifiers | |
Symbol | CyaB |
InterPro | IPR008173 |
CATH | 1YEM |
SCOP2 | 2ACA / SCOPe / SUPFAM |
CDD | cd07890 |
AC-IV was first reported in the bacterium Aeromonas hydrophila, and the structure of the AC-IV from Yersinia pestis has been reported. These are the smallest of the AC enzyme classes; the AC-IV (CyaB) from Yersinia is a dimer of 19 kDa subunits with no known regulatory components (PDB: 2FJT).[21] AC-IV forms a superfamily with mammalian thiamine-triphosphatase called CYTH (CyaB, thiamine triphosphatase).[22]
Classes V and VI
AC Class VI (DUF3095) | |||||||||
---|---|---|---|---|---|---|---|---|---|
Identifiers | |||||||||
Symbol | DUF3095 | ||||||||
Pfam | PF11294 | ||||||||
InterPro | IPR021445 | ||||||||
| |||||||||
contact prediction |
These forms of AC have been reported in specific bacteria (Prevotella ruminicola O68902 and Rhizobium etli Q8KY20, respectively) and have not been extensively characterized.[23] There are a few extra members (~400 in Pfam) known to be in class VI. Class VI enzymes possess a catalytic core similar to the one in Class III.[24]
Additional images
- Beta adrenergic receptor kinase pathway
References
- "PDB101: Molecule of the Month: G Proteins". RCSB: PDB-101. Retrieved 24 August 2020.
- Hancock, John (2010). Cell Signaling. pp. 189–195.
- Sadana R, Dessauer CW (February 2009). "Physiological roles for G protein-regulated adenylyl cyclase isoforms: insights from knockout and overexpression studies". Neuro-Signals. 17 (1): 5–22. doi:10.1159/000166277. PMC 2790773. PMID 18948702.
- Zhang G, Liu Y, Ruoho AE, Hurley JH (March 1997). "Structure of the adenylyl cyclase catalytic core". Nature. 386 (6622): 247–253. Bibcode:1997Natur.386..247Z. doi:10.1038/386247a0. PMID 9069282. S2CID 4329051.
- Linder JU (November 2008). "Structure-function relationships in Escherichia coli adenylate cyclase". The Biochemical Journal. 415 (3): 449–454. doi:10.1042/BJ20080350. PMID 18620542. (alignment)
- Ahuja N, Kumar P, Bhatnagar R (2004). "The adenylate cyclase toxins". Critical Reviews in Microbiology. 30 (3): 187–196. doi:10.1080/10408410490468795. PMID 15490970. S2CID 23893594.
- Khanppnavar B, Datta S (September 2018). "Crystal structure and substrate specificity of ExoY, a unique T3SS mediated secreted nucleotidyl cyclase toxin from Pseudomonas aeruginosa". Biochimica et Biophysica Acta (BBA) - General Subjects. 1862 (9): 2090–2103. doi:10.1016/j.bbagen.2018.05.021. PMID 29859257. S2CID 44151852.
- Guo Q, Shen Y, Lee YS, Gibbs CS, Mrksich M, Tang WJ (September 2005). "Structural basis for the interaction of Bordetella pertussis adenylyl cyclase toxin with calmodulin". The EMBO Journal. 24 (18): 3190–3201. doi:10.1038/sj.emboj.7600800. PMC 1224690. PMID 16138079.
- Drum CL, Yan SZ, Bard J, Shen YQ, Lu D, Soelaiman S, et al. (January 2002). "Structural basis for the activation of anthrax adenylyl cyclase exotoxin by calmodulin". Nature. 415 (6870): 396–402. Bibcode:2002Natur.415..396D. doi:10.1038/415396a. PMID 11807546. S2CID 773562.
- Reece J, Campbell N (2002). Biology. San Francisco: Benjamin Cummings. pp. 207. ISBN 978-0-8053-6624-2.
- Hanoune J, Defer N (April 2001). "Regulation and role of adenylyl cyclase isoforms". Annual Review of Pharmacology and Toxicology. 41 (1): 145–174. doi:10.1146/annurev.pharmtox.41.1.145. PMID 11264454.
- Schröder-Lang S, Schwärzel M, Seifert R, Strünker T, Kateriya S, Looser J, et al. (January 2007). "Fast manipulation of cellular cAMP level by light in vivo". Nature Methods. 4 (1): 39–42. doi:10.1038/nmeth975. PMID 17128267. S2CID 10616442.
- Linder JU, Schultz JE (December 2003). "The class III adenylyl cyclases: multi-purpose signalling modules". Cellular Signalling. 15 (12): 1081–1089. doi:10.1016/s0898-6568(03)00130-x. PMID 14575863.
- Rahman N, Buck J, Levin LR (November 2013). "pH sensing via bicarbonate-regulated "soluble" adenylyl cyclase (sAC)". Frontiers in Physiology. 4: 343. doi:10.3389/fphys.2013.00343. PMC 3838963. PMID 24324443.
- Hogan DA, Muhlschlegel FA (December 2011). "Candida albicans developmental regulation: adenylyl cyclase as a coincidence detector of parallel signals". Current Opinion in Microbiology. 14 (6): 682–686. doi:10.1016/j.mib.2011.09.014. PMID 22014725.
- Ostrom KF, LaVigne JE, Brust TF, Seifert R, Dessauer CW, Watts VJ, Ostrom RS (April 2022). "Physiological roles of mammalian transmembrane adenylyl cyclase isoforms". Physiological Reviews. 102 (2): 815–857. doi:10.1152/physrev.00013.2021. PMC 8759965. PMID 34698552.
- Esposito G, Jaiswal BS, Xie F, Krajnc-Franken MA, Robben TJ, Strik AM, et al. (March 2004). "Mice deficient for soluble adenylyl cyclase are infertile because of a severe sperm-motility defect". Proceedings of the National Academy of Sciences of the United States of America. 101 (9): 2993–2998. Bibcode:2004PNAS..101.2993E. doi:10.1073/pnas.0400050101. PMC 365733. PMID 14976244.
- Willoughby D, Cooper DM (July 2007). "Organization and Ca2+ regulation of adenylyl cyclases in cAMP microdomains". Physiological Reviews. 87 (3): 965–1010. CiteSeerX 10.1.1.336.3746. doi:10.1152/physrev.00049.2006. PMID 17615394.
- Mons N, Guillou JL, Jaffard R (April 1999). "The role of Ca2+/calmodulin-stimulable adenylyl cyclases as molecular coincidence detectors in memory formation". Cellular and Molecular Life Sciences. 55 (4): 525–533. doi:10.1007/s000180050311. PMID 10357223. S2CID 10849274.
- Neve KA, Seamans JK, Trantham-Davidson H (August 2004). "Dopamine receptor signaling". Journal of Receptor and Signal Transduction Research. 24 (3): 165–205. CiteSeerX 10.1.1.465.5011. doi:10.1081/RRS-200029981. PMID 15521361. S2CID 12407397.
- Gallagher DT, Smith NN, Kim SK, Heroux A, Robinson H, Reddy PT (September 2006). "Structure of the class IV adenylyl cyclase reveals a novel fold". Journal of Molecular Biology. 362 (1): 114–122. doi:10.1016/j.jmb.2006.07.008. PMID 16905149.
- Kohn G, Delvaux D, Lakaye B, Servais AC, Scholer G, Fillet M, et al. (2012). "High inorganic triphosphatase activities in bacteria and mammalian cells: identification of the enzymes involved". PLOS ONE. 7 (9): e43879. Bibcode:2012PLoSO...743879K. doi:10.1371/journal.pone.0043879. PMC 3440374. PMID 22984449.
- Cotta MA, Whitehead TR, Wheeler MB (July 1998). "Identification of a novel adenylate cyclase in the ruminal anaerobe, Prevotella ruminicola D31d". FEMS Microbiology Letters. 164 (2): 257–260. doi:10.1111/j.1574-6968.1998.tb13095.x. PMID 9682474. GenBank AF056932.
- Téllez-Sosa J, Soberón N, Vega-Segura A, Torres-Márquez ME, Cevallos MA (July 2002). "The Rhizobium etli cyaC product: characterization of a novel adenylate cyclase class". Journal of Bacteriology. 184 (13): 3560–3568. doi:10.1128/jb.184.13.3560-3568.2002. PMC 135151. PMID 12057950. GenBank AF299113.
Further reading
- Sodeman W, Sodeman T (2005). "Physiologic- and Adenylate Cyclase-Coupled Beta-Adrenergic Receptors". Sodeman's Pathologic Physiology: Mechanisms of Disease. W B Saunders Co. pp. 143–145. ISBN 978-0721610108.
External links

- Adenylyl Cyclases at the U.S. National Library of Medicine Medical Subject Headings (MeSH)
- Interactive 3D views of Adenylate cyclase at Proteopedia Adenylyl_cyclase