Vancomycin
Vancomycin is a glycopeptide antibiotic medication used to treat a number of bacterial infections.[6] It is recommended intravenously as a treatment for complicated skin infections, bloodstream infections, endocarditis, bone and joint infections, and meningitis caused by methicillin-resistant Staphylococcus aureus.[7] Blood levels may be measured to determine the correct dose.[8] Vancomycin is also taken by mouth as a treatment for severe Clostridium difficile colitis.[6] When taken by mouth it is poorly absorbed.[6]
![]() | |
![]() | |
Clinical data | |
---|---|
Pronunciation | /væŋkəˈmaɪsɪn/[1][2] |
Trade names | Vancocin, others[3] |
AHFS/Drugs.com | Monograph |
MedlinePlus | a604038 |
License data |
|
Pregnancy category |
|
Routes of administration | Intravenous, by mouth |
Drug class | Glycopeptide antibiotic |
ATC code | |
Legal status | |
Legal status | |
Pharmacokinetic data | |
Bioavailability | Negligible (by mouth) |
Metabolism | Excreted unchanged |
Elimination half-life | 4 h to 11 h (adults) 6 d to 10 d (adults, impaired renal function) |
Excretion | urine (IV), feces (by mouth) |
Identifiers | |
IUPAC name
| |
CAS Number | |
PubChem CID | |
PubChem SID | |
DrugBank | |
ChemSpider | |
UNII | |
KEGG | |
ChEBI | |
ChEMBL | |
CompTox Dashboard (EPA) | |
ECHA InfoCard | 100.014.338 |
Chemical and physical data | |
Formula | C66H75Cl2N9O24 |
Molar mass | 1449.27 g·mol−1 |
3D model (JSmol) | |
SMILES
| |
InChI
| |
(verify) |
Common side effects include pain in the area of injection and allergic reactions.[6] Occasionally, hearing loss, low blood pressure, or bone marrow suppression occur.[6] Safety in pregnancy is not clear, but no evidence of harm has been found,[6][9] and it is likely safe for use when breastfeeding.[10] It is a type of glycopeptide antibiotic and works by blocking the construction of a cell wall.[6]
Vancomycin was approved for medical use in the United States in 1958.[11] It is on the World Health Organization's List of Essential Medicines.[12][13] The World Health Organization classifies vancomycin as critically important for human medicine.[14] It is available as a generic medication.[8] Vancomycin is made by the soil bacterium Amycolatopsis orientalis.[6]
Medical uses
Vancomycin is indicated for the treatment of serious, life-threatening infections by Gram-positive bacteria unresponsive to other antibiotics.
The increasing emergence of vancomycin-resistant enterococci has resulted in the development of guidelines for use by the Centers for Disease Control Hospital Infection Control Practices Advisory Committee. These guidelines restrict use of vancomycin to these indications:[15][16]
- Treatment of serious infections caused by susceptible organisms resistant to penicillins (methicillin-resistant S. aureus (MRSA) and multidrug-resistant S. epidermidis (MRSE)) or in individuals with serious allergy to penicillins
- Treatment of pseudomembranous colitis caused by C. difficile; in particular, in cases of relapse or where the infection is unresponsive to metronidazole treatment (for this indication, vancomycin is given orally, rather than by its typical intravenous route)
- For treatment of infections caused by Gram-positive microorganisms in patients with serious allergies to beta-lactam antimicrobials.[16]
- Antibacterial prophylaxis for endocarditis following certain procedures in penicillin-hypersensitive individuals at high risk[16]
- Surgical prophylaxis for major procedures involving implantation of prostheses in institutions with a high rate of MRSA or MRSE[16]
- Early in treatment as an empiric antibiotic for possible MRSA infection while waiting for culture identification of the infecting organism
- Halting the progression of primary sclerosing cholangitis and preventing symptoms; vancomycin does not cure the patient and success is limited
- Treatment of endophthalmitis by intravitreal injection for gram-positive bacteria coverage.[17] It use to prevent the condition, however, is not recommended due to the risk of side effects.[18]
Spectrum of susceptibility
Vancomycin is considered a last resort medication for the treatment of sepsis and lower respiratory tract, skin, and bone infections caused by Gram-positive bacteria. The minimum inhibitory concentration susceptibility data for a few medically significant bacteria are:[19]
- S. aureus: 0.25 μg/mL to 4.0 μg/mL
- S. aureus (methicillin resistant or MRSA): 1 μg/mL to 138 μg/mL
- S. epidermidis: ≤0.12 μg/mL to 6.25 μg/mL
Side effects
Serum vancomycin levels may be monitored in an effort to reduce side effects, although the value of such monitoring has been questioned.[20] Peak and trough levels are usually monitored, and for research purposes, the area under the concentration curve is also sometimes used. Toxicity is best monitored by looking at trough values.[21]
Common adverse drug reactions (≥1% of patients) associated with IV vancomycin include: local pain, which may be severe, and thrombophlebitis.
Damage to the kidneys (nephrotoxicity) and to the hearing (ototoxicity) were side effects of the early impure versions of vancomycin, and these were prominent in the clinical trials conducted in the mid-1950s.[22][23] Later trials using purer forms of vancomycin found nephrotoxicity is an infrequent adverse effect (0.1% to 1% of patients), but this is accentuated in the presence of aminoglycosides.[24]
Rare adverse effects (<0.1% of patients) include: anaphylaxis, toxic epidermal necrolysis, erythema multiforme, red man syndrome, superinfection, thrombocytopenia, neutropenia, leukopenia, tinnitus, dizziness and/or ototoxicity, and DRESS syndrome.[25]
Vancomycin can induce platelet-reactive antibodies in the patient, leading to severe thrombocytopenia and bleeding with florid petechial hemorrhages, ecchymoses, and wet purpura.[26]
Vancomycin has traditionally been considered a nephrotoxic and ototoxic drug, based on observations by early investigators of elevated serum levels in renally impaired patients who had experienced ototoxicity, and subsequently through case reports in the medical literature. However, as the use of vancomycin increased with the spread of MRSA beginning in the 1970s, the previously reported rates of toxicity were recognized as not being observed. This was attributed to the removal of the impurities present in the earlier formulation of the drug, although those impurities were not specifically tested for toxicity.[22]
Nephrotoxicity
Subsequent reviews of accumulated case reports of vancomycin-related nephrotoxicity found many of the patients had also received other known nephrotoxins, in particular, aminoglycosides. Most of the rest had other confounding factors, or insufficient data regarding the possibility of such, that prohibited the clear association of vancomycin with the observed kidney dysfunction.
In 1994, the use of vancomycin monotherapy was clearly documented in only three of 82 available cases in the literature.[20] Prospective and retrospective studies attempting to evaluate the incidence of vancomycin-related nephrotoxicity have largely been methodologically flawed and have produced variable results. The most methodologically sound investigations indicate the actual incidence of vancomycin-induced nephrotoxicity is around 5% to 7%. To put this into context, similar rates of kidney dysfunction have been reported for cefamandole and benzylpenicillin, two reputedly non-nephrotoxic antibiotics.
In addition, evidence to relate nephrotoxicity to vancomycin serum levels is inconsistent. Some studies have indicated an increased rate of nephrotoxicity when trough levels exceed 10 µg/mL, but others have not reproduced these results. Nephrotoxicity has also been observed with concentrations within the "therapeutic" range, as well. In essence, the reputation of vancomycin as a nephrotoxin is overstated, and it has not been demonstrated that maintaining vancomycin serum levels within certain ranges will prevent its nephrotoxic effects, when they do occur.
Ototoxicity
Attempts to establish rates of vancomycin-induced ototoxicity are even more difficult due to the scarcity of quality evidence. The current consensus is that clearly related cases of vancomycin ototoxicity are rare. The association between vancomycin serum levels and ototoxicity is also uncertain. While cases of ototoxicity have been reported in patients whose vancomycin serum level exceeded 80 µg/mL, cases have been reported in patients with therapeutic levels, as well. Thus, whether therapeutic drug monitoring of vancomycin for the purpose of maintaining "therapeutic" levels will prevent ototoxicity also remains unproven.
Interactions with other nephrotoxins
Another area of controversy and uncertainty concerns the question of whether, and if so, to what extent, vancomycin increases the toxicity of other nephrotoxins. Clinical studies have yielded variable results, but animal models indicate some increased nephrotoxic effect probably occurs when vancomycin is added to nephrotoxins such as aminoglycosides. However, a dose- or serum level-effect relationship has not been established.
Dosing considerations
The recommended parenteral dosage in adults is 500 mg iv every 6 hours or 1000 mg every 12 hours, with modification to achieve a therapeutic range as needed. The recommended oral dosage in the treatment of antibiotic induced pseudomembranous enterocolitis is 125 to 500 mg every 6 hours for 7 to 10 days.[27]
Intravenous vs oral administration
Vancomycin must be given intravenously (IV) for systemic therapy, since it is not absorbed from the intestine. It is a large hydrophilic molecule that partitions poorly across the gastrointestinal mucosa. Due to short half-life, it is often injected twice daily.[28]
The only approved indication for oral vancomycin therapy is in the treatment of pseudomembranous colitis, where it must be given orally to reach the site of infection in the colon. Following oral administration, the fecal concentration of vancomycin is around 500 µg/mL[29] (sensitive strains of C. difficile have a mean inhibitory concentration of ≤2 µg/mL[30])
Inhaled vancomycin has also been used (off-label), via nebulizer, for treatment of various infections of the upper and lower respiratory tract.
There is an ongoing debate as to whether vancomycin should be given through a central or peripheral line. According to a 2014 review, midline catheters are a safe option for administration.[31]
Vancomycin Flushing Reaction (aka "Red man syndrome")
Vancomycin is recommended to be administered in a dilute solution slowly, over at least 60 min (maximum rate of 10 mg/min for doses >500 mg)[15] due to the high incidence of pain and thrombophlebitis and to avoid an infusion reaction known as vancomycin flushing reaction. This phenomenon has been often clinically referred to as "red man syndrome". The reaction usually appears within 4 to 10 min after the commencement or soon after the completion of an infusion and is characterized by flushing and/or an erythematous rash that affects the face, neck, and upper torso, attributed due to release of histamine from the mast cells. These findings are due to interaction of vancomycin with MRGPRX2, a GPCR mediating IgE-independent mast cell degranulation.[32] Less frequently, hypotension and angioedema may also occur. Symptoms may be treated or prevented with antihistamines, including diphenhydramine, and are less likely to occur with slow infusion.[33][34]
Therapeutic drug monitoring
Plasma level monitoring of vancomycin is necessary due to the drug's biexponential distribution, intermediate hydrophilicity, and potential for ototoxicity and nephrotoxicity, especially in populations with poor renal function and/or increased propensity to bacterial infection. Vancomycin activity is considered to be time-dependent; that is, antimicrobial activity depends on the duration that the serum drug concentration exceeds the minimum inhibitory concentration of the target organism. Thus, peak serum levels have not been shown to correlate with efficacy or toxicity; indeed, concentration monitoring is unnecessary in most cases. Circumstances in which therapeutic drug monitoring is warranted include: patients receiving concomitant aminoglycoside therapy, patients with (potentially) altered pharmacokinetic parameters, patients on haemodialysis, patients administered high-dose or prolonged treatment, and patients with impaired renal function. In such cases, trough concentrations are measured.[15][20][35][36]
Target ranges for serum vancomycin concentrations have changed over the years. Early authors suggested peak levels of 30 to 40 mg/L and trough levels of 5 to 10 mg/L,[37] but current recommendations are that peak levels need not be measured and that trough levels of 10 to 15 mg/L or 15 to 20 mg/L, depending on the nature of the infection and the specific needs of the patient, may be appropriate.[38][39] Using measured vancomycin concentrations to calculate doses optimizes therapy in patients with augmented renal clearance.[40]
Biosynthesis
Vancomycin is made by the soil bacterium Amycolatopsis orientalis.[6]
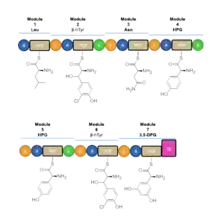
Vancomycin biosynthesis occurs primarily via three nonribosomal protein syntheses (NRPSs) VpsA, VpsB, and VpsC.[41] The enzymes determine the amino acid sequence during its assembly through its 7 modules. Before vancomycin is assembled through NRPS, the non-proteinogenic amino acids are first synthesized. L-tyrosine is modified to become the β-hydroxytyrosine (β-HT) and 4-hydroxyphenylglycine (4-Hpg) residues. 3,5 dihydroxyphenylglycine ring (3,5-DPG) is derived from acetate.[42]
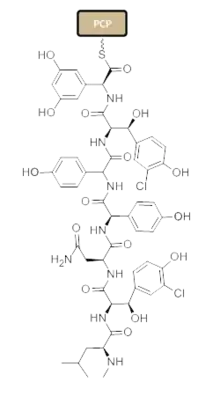
Nonribosomal peptide synthesis occurs through distinct modules that can load and extend the protein by one amino acid per module through the amide bond formation at the contact sites of the activating domains.[43] Each module typically consists of an adenylation (A) domain, a peptidyl carrier protein (PCP) domain, and a condensation (C) domain. In the A domain, the specific amino acid is activated by converting into an aminoacyl adenylate enzyme complex attached to a 4'phosphopantetheine cofactor by thioesterification[44][45] The complex is then transferred to the PCP domain with the expulsion of AMP. The PCP domain uses the attached 4'-phosphopantethein prosthetic group to load the growing peptide chain and their precursors.[46] The organization of the modules necessary to biosynthesize Vancomycin is shown in Figure 1. In the biosynthesis of Vancomycin, additional modification domains are present, such as the epimerization (E) domain, which isomerizes the amino acid from one stereochemistry to another, and a thioesterase domain (TE) is used as a catalyst for cyclization and releases of the molecule via a thioesterase scission.
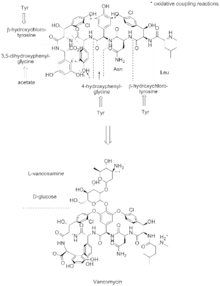
A set of NRPS enzymes (peptide synthase VpsA, VpsB, and VpsC) are responsible for assembling the heptapeptide. (Figure 2).[43] VpsA codes for modules 1, 2, and 3. VpsB codes for modules 4, 5, and 6, and VpsC codes for module 7. The vancomycin aglycone contains 4 D-amino acids, although the NRPSs only contain 3 epimerization domains. The origin of D-Leu at residue 1 is not known. The three peptide syntheses are located at the start of the region of the bacterial genome linked with antibiotic biosynthesis, and span 27 kb.[43]
β-hydroxytyrosine (β-HT) is synthesized prior to incorporation into the heptapeptide backbone. L-tyrosine is activated and loaded on the NRPS VpsD, hydroxylated by OxyD, and released by the thioesterase Vhp.[47] The timing of the chlorination by halogenase VhaA during biosynthesis is currently undetermined, but is proposed to occur before the complete assembly of the heptapeptide.[48]
After the linear heptapeptide molecule is synthesized, vancomycin has to undergo further modifications, such as oxidative cross-linking and glycosylation, in trans by distinct enzymes, referred to as tailoring enzymes, to become biologically active (Figure 3). To convert the linear heptapeptide to cross-linked, glycosylated vancomycin, six enzymes, are required. The enzymes OxyA, OxyB, OxyC, and OxyD are cytochrome P450 enzymes. OxyB catalyzes oxidative cross-linking between residues 4 and 6, OxyA between residues 2 and 4, and OxyC between residues 5 and 7. This cross-linking occurs while the heptapeptide is covalently bound to the PCP domain of the 7th NRPS module. These P450s are recruited by the X domain present in the 7th NRPS module, which is unique to glycopeptide antibiotic biosynthesis.[49] The cross-linked heptapeptide is then released by the action of the TE domain, and methyltransferase Vmt then N-methylates the terminal leucine residue. GtfE then joins D-glucose to the phenolic oxygen of residue 4, followed by the addition of vancosamine catalyzed by GtfD.
Some of the glycosyltransferases capable of glycosylating vancomycin and related nonribosomal peptides display notable permissivity and have been employed for generating libraries of differentially glycosylated analogs through a process known as glycorandomization.[50][51][52]
Total synthesis
Both the vancomycin aglycone[53][54] and the complete vancomycin molecule[55] have been targets successfully reached by total synthesis. The target was first achieved by David Evans in October 1998, KC Nicolaou in December 1998, Dale Boger in 1999, and has recently been more selectively synthesized again by Dale Boger in 2020.
Pharmacology and chemistry
Vancomycin is a branched tricyclic glycosylated nonribosomal peptide produced by the Actinomycetota species Amycolatopsis orientalis (formerly designated Nocardia orientalis).
Vancomycin exhibits atropisomerism — it has multiple chemically distinct rotamers owing to the rotational restriction of some of the bonds. The form present in the drug is the thermodynamically more stable conformer.
Mechanism of action
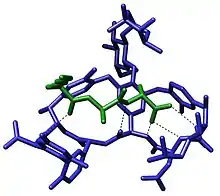
Vancomycin acts by inhibiting proper cell wall synthesis in Gram-positive bacteria. Due to the different mechanism by which Gram-negative bacteria produce their cell walls and the various factors related to entering the outer membrane of Gram-negative organisms, vancomycin is not active against them (except some nongonococcal species of Neisseria).
The large hydrophilic molecule is able to form hydrogen bond interactions with the terminal D-alanyl-D-alanine moieties of the NAM/NAG-peptides. Under normal circumstances, this is a five-point interaction. This binding of vancomycin to the D-Ala-D-Ala prevents cell wall synthesis of the long polymers of N-acetylmuramic acid (NAM) and N-acetylglucosamine (NAG) that form the backbone strands of the bacterial cell wall, and it prevents the backbone polymers that do manage to form from cross-linking with each other.[57]
Plant tissue culture
Vancomycin is one of the few antibiotics used in plant tissue culture to eliminate Gram-positive bacterial infection. It has relatively low toxicity to plants.[58][59]
Antibiotic resistance
Intrinsic resistance
A few Gram-positive bacteria are intrinsically resistant to vancomycin: Leuconostoc and Pediococcus species, but these organisms rarely cause diseases in humans.[60] Most Lactobacillus species are also intrinsically resistant to vancomycin,[60] with the exception of L. acidophilus and L. delbrueckii, which are sensitive.[61] Other Gram-positive bacteria with intrinsic resistance to vancomycin include Erysipelothrix rhusiopathiae, Weissella confusa, and Clostridium innocuum.[62][63][64]
Most Gram-negative bacteria are intrinsically resistant to vancomycin because their outer membranes are impermeable to large glycopeptide molecules[65] (with the exception of some non-gonococcal Neisseria species).[66]
Acquired resistance
Evolution of microbial resistance to vancomycin is a growing problem, in particular, within healthcare facilities such as hospitals. While newer alternatives to vancomycin exist, such as linezolid (2000) and daptomycin (2003), the widespread use of vancomycin makes resistance to the drug a significant worry, especially for individual patients if resistant infections are not quickly identified and the patient continues the ineffective treatment. Vancomycin-resistant Enterococcus emerged in 1986.[67] Vancomycin resistance evolved in more common pathogenic organisms during the 1990s and 2000s, including vancomycin-intermediate S. aureus (VISA) and vancomycin-resistant S. aureus (VRSA).[68][69] Agricultural use of avoparcin, another similar glycopeptide antibiotic, may have contributed to the evolution of vancomycin-resistant organisms.[70][71][72][73]
One mechanism of resistance to vancomycin involves the alteration to the terminal amino acid residues of the NAM/NAG-peptide subunits, under normal conditions, D-alanyl-D-alanine, to which vancomycin binds. The D-alanyl-D-lactate variation results in the loss of one hydrogen-bonding interaction (4, as opposed to 5 for D-alanyl-D-alanine) possible between vancomycin and the peptide. This loss of just one point of interaction results in a 1000-fold decrease in affinity. The D-alanyl-D-serine variation causes a six-fold loss of affinity between vancomycin and the peptide, likely due to steric hindrance.[74]
In enterococci, this modification appears to be due to the expression of an enzyme that alters the terminal residue. Three main resistance variants have been characterised to date among resistant Enterococcus faecium and E. faecalis populations:
- VanA - enterococcal resistance to vancomycin and teicoplanin; inducible on exposure to these agents
- VanB - lower-level enterococcal resistance; inducible by vancomycin, but strains may remain susceptible to teicoplanin
- VanC - least clinically important; enterococci resistant only to vancomycin; constitutive resistance
Variant of vancomycin has been tested that binds to the resistant D-lactic acid variation in vancomycin-resistant bacterial cell walls, and also binds well to the original target (vancomycin-susceptible bacteria).[75][76]
"Re-gained" vancomycin
In 2020 a team at the University Hospital Heidelberg (Germany) re-gained the antibacterial power of vancomycin by modifying the molecule with a cationic oligopeptide. The oligopeptide consists of six arginin units in Position VN. In comparison to the unmodified vancomycin the activity against vancomycin-resistant bacteria could be enhanced by a factor of 1 000.[77][78] This pharmacon is still in preclinical development. Therefore, a potential approval will take several more years.
History
Vancomycin was first isolated in 1953 by Edmund Kornfeld (working at Eli Lilly) from a soil sample collected from the interior jungles of Borneo by a missionary, Rev. William M. Bouw (1918-2006).[79] The organism that produced it was eventually named Amycolatopsis orientalis.[22] The original indication for vancomycin was for the treatment of penicillin-resistant Staphylococcus aureus.[22][23]
The compound was initially called compound 05865, but was eventually given the generic name vancomycin, derived from the term "vanquish".[22] One advantage that was quickly apparent was that staphylococci did not develop significant resistance, despite serial passage in culture media containing vancomycin. The rapid development of penicillin resistance by staphylococci led to its being fast-tracked for approval by the Food and Drug Administration. In 1958, Eli Lilly first marketed vancomycin hydrochloride under the trade name Vancocin.[23]
Vancomycin never became the first-line treatment for S. aureus for several reasons:
- It possesses poor oral bioavailability, so must be given intravenously for most infections.
- β-Lactamase-resistant semisynthetic penicillins such as methicillin (and its successors, nafcillin and cloxacillin) were subsequently developed, which have better activity against non-MRSA staphylococci.
- Early trials used early, impure forms of the drug ("Mississippi mud"), which were found to be toxic to the inner ear and to the kidneys;[80] these findings led to vancomycin's being relegated to the position of a drug of last resort.[23]
In 2004, Eli Lilly licensed Vancocin to ViroPharma in the U.S., Flynn Pharma in the UK, and Aspen Pharmacare in Australia. The patent had expired in the early 1980s, and the FDA authorized the sale of several generic versions in the US, including from manufacturers Bioniche Pharma, Baxter Healthcare, Sandoz, Akorn-Strides, and Hospira.[81]
References
- "vancomycin". Merriam-Webster Dictionary.
- "vancomycin - definition of vancomycin in English from the Oxford dictionary". OxfordDictionaries.com. Archived from the original on 20 September 2013. Retrieved 20 January 2016.
- "Vancomycin". Drugs.com. 2 December 2019. Retrieved 24 December 2019.
- "Vancomycin Use During Pregnancy". Drugs.com. 27 March 2019. Retrieved 24 December 2019.
- https://www.ema.europa.eu/documents/psusa/vancomycin-list-nationally-authorised-medicinal-products-psusa/00003097/202001_en.pdf
- "Vancocin". The American Society of Health-System Pharmacists. Archived from the original on 6 September 2015. Retrieved 4 September 2015.
- Liu C, Bayer A, Cosgrove SE, Daum RS, Fridkin SK, Gorwitz RJ, et al. (February 2011). "Clinical practice guidelines by the infectious diseases society of america for the treatment of methicillin-resistant Staphylococcus aureus infections in adults and children: executive summary". Clinical Infectious Diseases. 52 (3): 285–92. doi:10.1093/cid/cir034. PMID 21217178.
- Hamilton R (2015). Tarascon Pocket Pharmacopoeia 2015 Deluxe Lab-Coat Edition. Jones & Bartlett Learning. p. 91. ISBN 9781284057560.
- "Prescribing medicines in pregnancy database". Australian Government. September 2015. Archived from the original on 8 April 2014.
- "Vancomycin use while Breastfeeding". Archived from the original on 7 September 2015. Retrieved 5 September 2015.
- Levine DP (January 2006). "Vancomycin: a history". Clinical Infectious Diseases. 42 Suppl 1: S5–12. doi:10.1086/491709. PMID 16323120.
- World Health Organization (2019). World Health Organization model list of essential medicines: 21st list 2019. Geneva: World Health Organization. hdl:10665/325771. WHO/MVP/EMP/IAU/2019.06. License: CC BY-NC-SA 3.0 IGO.
- World Health Organization (2021). World Health Organization model list of essential medicines: 22nd list (2021). Geneva: World Health Organization. hdl:10665/345533. WHO/MHP/HPS/EML/2021.02.
- World Health Organization (2019). Critically important antimicrobials for human medicine (6th revision ed.). Geneva: World Health Organization. hdl:10665/312266. ISBN 9789241515528.
- Rossi S, ed. (2006). Australian Medicines Handbook. Adelaide: Australian Medicines Handbook. ISBN 0-9757919-2-3.
- "Recommendations for preventing the spread of vancomycin resistance. Recommendations of the Hospital Infection Control Practices Advisory Committee (HICPAC)". MMWR. Recommendations and Reports. 44 (RR-12): 1–13. September 1995. PMID 7565541. Archived from the original on 23 September 2006.
- Lifshitz T, Lapid-Gortzak R, Finkelman Y, Klemperer I (January 2000). "Vancomycin and ceftazidime incompatibility upon intravitreal injection". The British Journal of Ophthalmology. 84 (1): 117–8. doi:10.1136/bjo.84.1.117a. PMC 1723217. PMID 10691328.
- Office of the Commissioner. "Safety Alerts for Human Medical Products - Intraocular Injections of a Compounded Triamcinolone, Moxifloxacin, and Vancomycin (TMV) Formulation: FDA Statement - Case of Hemorrhagic Occlusive Retinal Vasculitis". www.fda.gov. Retrieved 6 October 2017.
- "Vancomycin (Vancocyn, Lyphocin) | the Antimicrobial Index Knowledgebase - TOKU-E". Archived from the original on 27 February 2014. Retrieved 26 February 2014.
- Cantú TG, Yamanaka-Yuen NA, Lietman PS (April 1994). "Serum vancomycin concentrations: reappraisal of their clinical value". Clinical Infectious Diseases. 18 (4): 533–43. doi:10.1093/clinids/18.4.533. PMID 8038306.
- Lodise TP, Patel N, Lomaestro BM, Rodvold KA, Drusano GL (August 2009). "Relationship between initial vancomycin concentration-time profile and nephrotoxicity among hospitalized patients". Clinical Infectious Diseases. 49 (4): 507–14. doi:10.1086/600884. PMID 19586413.
- Levine DP (January 2006). "Vancomycin: a history". Clinical Infectious Diseases. 42 (Suppl 1): S5-12. doi:10.1086/491709. PMID 16323120.
- Moellering RC (January 2006). "Vancomycin: a 50-year reassessment". Clinical Infectious Diseases. 42 (Suppl 1): S3-4. doi:10.1086/491708. PMID 16323117.
- Farber BF, Moellering RC (January 1983). "Retrospective study of the toxicity of preparations of vancomycin from 1974 to 1981". Antimicrobial Agents and Chemotherapy. 23 (1): 138–41. doi:10.1128/AAC.23.1.138. PMC 184631. PMID 6219616.
- Blumenthal KG, Patil SU, Long AA (1 April 2012). "The importance of vancomycin in drug rash with eosinophilia and systemic symptoms (DRESS) syndrome". Allergy and Asthma Proceedings. 33 (2): 165–71. doi:10.2500/aap.2012.33.3498. PMID 22525393.
- Von Drygalski A, Curtis BR, Bougie DW, McFarland JG, Ahl S, Limbu I, et al. (March 2007). "Vancomycin-induced immune thrombocytopenia". The New England Journal of Medicine. 356 (9): 904–10. doi:10.1056/NEJMoa065066. PMID 17329697.
- Vancomycin, Bethesda (MD): National Institute of Diabetes and Digestive and Kidney Diseases, 2012, PMID 31644188, retrieved 25 February 2021
Text was copied from this source, which is available under a Creative Commons Attribution 4.0 International License.
- Van Bambeke F (August 2006). "Glycopeptides and glycodepsipeptides in clinical development: a comparative review of their antibacterial spectrum, pharmacokinetics and clinical efficacy". Current Opinion in Investigational Drugs. 7 (8): 740–9. PMID 16955686.
- Edlund C, Barkholt L, Olsson-Liljequist B, Nord CE (September 1997). "Effect of vancomycin on intestinal flora of patients who previously received antimicrobial therapy". Clinical Infectious Diseases. 25 (3): 729–32. doi:10.1086/513755. PMID 9314469.
- Peláez T, Alcalá L, Alonso R, Rodríguez-Créixems M, García-Lechuz JM, Bouza E (June 2002). "Reassessment of Clostridium difficile susceptibility to metronidazole and vancomycin". Antimicrobial Agents and Chemotherapy. 46 (6): 1647–50. doi:10.1128/AAC.46.6.1647-1650.2002. PMC 127235. PMID 12019070.
- Moureau NL (24 February 2021). "Is the pH of vancomycin an indication for central venous access?". The Journal of Vascular Access. 15 (4): 249–250. doi:10.5301/jva.5000223. PMC 6159816. PMID 24811587.
- Azimi E, Reddy VB, Lerner EA (March 2017). "Brief communication: MRGPRX2, atopic dermatitis and red man syndrome". Itch. 2 (1): e5. doi:10.1097/itx.0000000000000005. PMC 5375112. PMID 28367504.
- Sivagnanam S, Deleu D (April 2003). "Red man syndrome". Critical Care. 7 (2): 119–20. doi:10.1186/cc1871. PMC 270616. PMID 12720556.
- James W, Berger T, Elston D (2005). Andrews' Diseases of the Skin: Clinical Dermatology (10th ed.). Saunders. pp. 120–1. ISBN 0-7216-2921-0.
- Moellering RC (April 1994). "Monitoring serum vancomycin levels: climbing the mountain because it is there?". Clinical Infectious Diseases. 18 (4): 544–6. doi:10.1093/clinids/18.4.544. PMID 8038307.
- Karam CM, McKinnon PS, Neuhauser MM, Rybak MJ (March 1999). "Outcome assessment of minimizing vancomycin monitoring and dosing adjustments". Pharmacotherapy. 19 (3): 257–66. doi:10.1592/phco.19.4.257.30933. PMID 10221365. S2CID 24947921.
- Geraci JE (October 1977). "Vancomycin". Mayo Clinic Proceedings. 52 (10): 631–4. PMID 909314.
- Rybak M, Lomaestro B, Rotschafer JC, Moellering R, Craig W, Billeter M, et al. (January 2009). "Therapeutic monitoring of vancomycin in adult patients: a consensus review of the American Society of Health-System Pharmacists, the Infectious Diseases Society of America, and the Society of Infectious Diseases Pharmacists". American Journal of Health-System Pharmacy. 66 (1): 82–98. doi:10.2146/ajhp080434. PMID 19106348. S2CID 11692065.
- Thomson AH, Staatz CE, Tobin CM, Gall M, Lovering AM (May 2009). "Development and evaluation of vancomycin dosage guidelines designed to achieve new target concentrations". The Journal of Antimicrobial Chemotherapy. 63 (5): 1050–7. doi:10.1093/jac/dkp085. PMID 19299472.
- Izumisawa T, Kaneko T, Soma M, Imai M, Wakui N, Hasegawa H, et al. (December 2019). "Augmented Renal Clearance of Vancomycin in Hematologic Malignancy Patients". Biological & Pharmaceutical Bulletin. 42 (12): 2089–2094. doi:10.1248/bpb.b19-00652. PMID 31534058.
- Samel SA, Marahiel MA, Essen LO (May 2008). "How to tailor non-ribosomal peptide products--new clues about the structures and mechanisms of modifying enzymes". Molecular BioSystems. 4 (5): 387–93. doi:10.1039/b717538h. PMID 18414736.
- Dewick PM (2002). Medicinal natural products: a biosynthetic approach. New York: Wiley. ISBN 978-0-471-49641-0.
- van Wageningen AM, Kirkpatrick PN, Williams DH, Harris BR, Kershaw JK, Lennard NJ, et al. (March 1998). "Sequencing and analysis of genes involved in the biosynthesis of a vancomycin group antibiotic". Chemistry & Biology. 5 (3): 155–62. doi:10.1016/S1074-5521(98)90060-6. PMID 9545426.
- Schlumbohm W, Stein T, Ullrich C, Vater J, Krause M, Marahiel MA, et al. (December 1991). "An active serine is involved in covalent substrate amino acid binding at each reaction center of gramicidin S synthetase". The Journal of Biological Chemistry. 266 (34): 23135–41. doi:10.1016/S0021-9258(18)54473-2. PMID 1744112.
- Stein T, Vater J, Kruft V, Otto A, Wittmann-Liebold B, Franke P, et al. (June 1996). "The multiple carrier model of nonribosomal peptide biosynthesis at modular multienzymatic templates". The Journal of Biological Chemistry. 271 (26): 15428–35. doi:10.1074/jbc.271.26.15428. PMID 8663196.
- Kohli RM, Walsh CT, Burkart MD (August 2002). "Biomimetic synthesis and optimization of cyclic peptide antibiotics". Nature. 418 (6898): 658–61. Bibcode:2002Natur.418..658K. doi:10.1038/nature00907. PMID 12167866. S2CID 4380296.
- Puk O, Bischoff D, Kittel C, Pelzer S, Weist S, Stegmann E, et al. (September 2004). "Biosynthesis of chloro-beta-hydroxytyrosine, a nonproteinogenic amino acid of the peptidic backbone of glycopeptide antibiotics". Journal of Bacteriology. 186 (18): 6093–100. doi:10.1128/JB.186.18.6093-6100.2004. PMC 515157. PMID 15342578.
- Schmartz PC, Zerbe K, Abou-Hadeed K, Robinson JA (August 2014). "Bis-chlorination of a hexapeptide-PCP conjugate by the halogenase involved in vancomycin biosynthesis". Organic & Biomolecular Chemistry. 12 (30): 5574–7. doi:10.1039/C4OB00474D. PMID 24756572.
- Haslinger K, Peschke M, Brieke C, Maximowitsch E, Cryle MJ (May 2015). "X-domain of peptide synthetases recruits oxygenases crucial for glycopeptide biosynthesis". Nature. 521 (7550): 105–9. Bibcode:2015Natur.521..105H. doi:10.1038/nature14141. PMID 25686610. S2CID 4466657.
- Fu X, Albermann C, Jiang J, Liao J, Zhang C, Thorson JS (December 2003). "Antibiotic optimization via in vitro glycorandomization". Nature Biotechnology. 21 (12): 1467–9. doi:10.1038/nbt909. PMID 14608364. S2CID 2469387.
- Fu X, Albermann C, Zhang C, Thorson JS (April 2005). "Diversifying vancomycin via chemoenzymatic strategies". Organic Letters. 7 (8): 1513–5. doi:10.1021/ol0501626. PMID 15816740.
- Peltier-Pain P, Marchillo K, Zhou M, Andes DR, Thorson JS (October 2012). "Natural product disaccharide engineering through tandem glycosyltransferase catalysis reversibility and neoglycosylation". Organic Letters. 14 (19): 5086–9. doi:10.1021/ol3023374. PMC 3489467. PMID 22984807.
- Evans DA, Wood MR, Trotter BW, Richardson TI, Barrow JC, Katz JL (October 1998). "Total Syntheses of Vancomycin and Eremomycin Aglycons". Angewandte Chemie. 37 (19): 2700–2704. doi:10.1002/(SICI)1521-3773(19981016)37:19<2700::AID-ANIE2700>3.0.CO;2-P. PMID 29711601.
- Herzner H, Rück-Braun K (2008). "38. Crossing the Finishing Line: Total Syntheses of the Vancomycin Aglycon". In Schmalz HG (ed.). Organic Synthesis Highlights. Vol. IV. John Wiley & Sons. pp. 281–288. doi:10.1002/9783527619979.ch38. ISBN 9783527619979.
- Nicolaou KC, Mitchell HJ, Jain NF, Winssinger N, Hughes R, Bando T (1999). "Total Synthesis of Vancomycin". Angew. Chem. Int. Ed. 38 (1–2): 240–244. doi:10.1002/(SICI)1521-3773(19990115)38:1/2<240::AID-ANIE240>3.0.CO;2-5.
- Knox JR, Pratt RF (July 1990). "Different modes of vancomycin and D-alanyl-D-alanine peptidase binding to cell wall peptide and a possible role for the vancomycin resistance protein". Antimicrobial Agents and Chemotherapy. 34 (7): 1342–7. doi:10.1128/AAC.34.7.1342. PMC 175978. PMID 2386365.
- Clinical Pharmacology
- vancomcin for plant cell culture Archived 2012-05-04 at the Wayback Machine
- Pazuki A, Asghari J, Sohani MM, Pessarakli M, Aflaki F (2014). "Effects of Some Organic Nitrogen Sources and Antibiotics on Callus Growth of Indica Rice Cultivars". Journal of Plant Nutrition. 38 (8): 1231–1240. doi:10.1080/01904167.2014.983118. S2CID 84495391.
- Swenson JM, Facklam RR, Thornsberry C (April 1990). "Antimicrobial susceptibility of vancomycin-resistant Leuconostoc, Pediococcus, and Lactobacillus species". Antimicrobial Agents and Chemotherapy. 34 (4): 543–9. doi:10.1128/AAC.34.4.543. PMC 171641. PMID 2344161.
- Hamilton-Miller JM, Shah S (February 1998). "Vancomycin susceptibility as an aid to the identification of lactobacilli". Letters in Applied Microbiology. 26 (2): 153–4. doi:10.1046/j.1472-765X.1998.00297.x. PMID 9569701. S2CID 221924592.
- Romney M, Cheung S, Montessori V (July 2001). "Erysipelothrix rhusiopathiae endocarditis and presumed osteomyelitis". The Canadian Journal of Infectious Diseases. 12 (4): 254–6. doi:10.1155/2001/912086. PMC 2094827. PMID 18159347.
- David V, Bozdogan B, Mainardi JL, Legrand R, Gutmann L, Leclercq R (June 2004). "Mechanism of intrinsic resistance to vancomycin in Clostridium innocuum NCIB 10674". Journal of Bacteriology. 186 (11): 3415–22. doi:10.1128/JB.186.11.3415-3422.2004. PMC 415764. PMID 15150227.
- Kumar A, Augustine D, Sudhindran S, Kurian AM, Dinesh KR, Karim S, Philip R (October 2011). "Weissella confusa: a rare cause of vancomycin-resistant Gram-positive bacteraemia". Journal of Medical Microbiology. 60 (Pt 10): 1539–1541. doi:10.1099/jmm.0.027169-0. PMID 21596906.
- Quintiliani Jr R, Courvalin P (1995). "Mechanisms of Resistance to Antimicrobial Agents". In Murray PR, Baron EJ, Pfaller MA, Tenover FC, Yolken RH (eds.). Manual of Clinical Microbiology (6th ed.). Washington DC: ASM Press. pp. 1319. ISBN 978-1-55581-086-3.
- Geraci JE, Wilson WR (1981). "Vancomycin therapy for infective endocarditis". Reviews of Infectious Diseases. 3 (suppl): S250-8. doi:10.1093/clinids/3.Supplement_2.S250. PMID 7342289.
- Murray BE (March 2000). "Vancomycin-resistant enterococcal infections". The New England Journal of Medicine. 342 (10): 710–21. doi:10.1056/NEJM200003093421007. PMID 10706902.
The first reports of vancomycin-resistant enterococci (later classified as VanA type of resistance) involved strains of E. faecium that were resistant to vancomycin and teicoplanin (another glycopeptide) and that were isolated from patients in France and England in 1986. Vancomycin-resistant E. faecalis, subsequently classified as VanB type, was recovered from patients in Missouri in 1987.
- Smith TL, Pearson ML, Wilcox KR, Cruz C, Lancaster MV, Robinson-Dunn B, et al. (February 1999). "Emergence of vancomycin resistance in Staphylococcus aureus. Glycopeptide-Intermediate Staphylococcus aureus Working Group". The New England Journal of Medicine. 340 (7): 493–501. doi:10.1056/NEJM199902183400701. PMID 10021469.
- McDonald LC, Killgore GE, Thompson A, Owens RC, Kazakova SV, Sambol SP, et al. (December 2005). "An epidemic, toxin gene-variant strain of Clostridium difficile". The New England Journal of Medicine. 353 (23): 2433–41. doi:10.1056/NEJMoa051590. PMID 16322603. S2CID 43628397.
- Acar J, Casewell M, Freeman J, Friis C, Goossens H (September 2000). "Avoparcin and virginiamycin as animal growth promoters: a plea for science in decision-making". Clinical Microbiology and Infection. 6 (9): 477–82. doi:10.1046/j.1469-0691.2000.00128.x. PMID 11168181.
- Bager F, Madsen M, Christensen J, Aarestrup FM (July 1997). "Avoparcin used as a growth promoter is associated with the occurrence of vancomycin-resistant Enterococcus faecium on Danish poultry and pig farms". Preventive Veterinary Medicine. 31 (1–2): 95–112. doi:10.1016/S0167-5877(96)01119-1. PMID 9234429. S2CID 4958557.
- Collignon PJ (August 1999). "Vancomycin-resistant enterococci and use of avoparcin in animal feed: is there a link?". The Medical Journal of Australia. 171 (3): 144–6. doi:10.5694/j.1326-5377.1999.tb123568.x. PMID 10474607. S2CID 24378463.
- Lauderdale TL, Shiau YR, Wang HY, Lai JF, Huang IW, Chen PC, et al. (March 2007). "Effect of banning vancomycin analogue avoparcin on vancomycin-resistant enterococci in chicken farms in Taiwan" (PDF). Environmental Microbiology. 9 (3): 819–23. doi:10.1111/j.1462-2920.2006.01189.x. PMID 17298380.
- Pootoolal J, Neu J, Wright GD (2002). "Glycopeptide antibiotic resistance". Annual Review of Pharmacology and Toxicology. 42: 381–408. doi:10.1146/annurev.pharmtox.42.091601.142813. PMID 11807177.
- Xie J, Pierce JG, James RC, Okano A, Boger DL (September 2011). "A redesigned vancomycin engineered for dual D-Ala-D-ala And D-Ala-D-Lac binding exhibits potent antimicrobial activity against vancomycin-resistant bacteria". Journal of the American Chemical Society. 133 (35): 13946–9. doi:10.1021/ja207142h. PMC 3164945. PMID 21823662.
- Okano A, Isley NA, Boger DL (June 2017). "4]vancomycin with added synergistic mechanisms of action provide durable and potent antibiotics". Proceedings of the National Academy of Sciences of the United States of America. 114 (26): E5052–E5061. doi:10.1073/pnas.1704125114. PMC 5495262. PMID 28559345.
- Umstätter F, Domhan C, Hertlein T, Ohlsen K, Mühlberg E, Kleist C, et al. (June 2020). "Vancomycin Resistance Is Overcome by Conjugation of Polycationic Peptides". Angewandte Chemie. 59 (23): 8823–8827. doi:10.1002/anie.202002727. PMC 7323874. PMID 32190958.
- EP 3846854A2, Mier W, Umstätter F, Uhl P, Domhan C, "Improved polypeptide coupled antibiotics."
- Shnayerson M, Plotkin M (2003). The Killers Within: The Deadly Rise of Drug-Resistant Bacteria. Back Bay Books. ISBN 978-0-316-73566-7.
- Griffith RS (1981). "Introduction to vancomycin". Reviews of Infectious Diseases. 3 suppl: S200-4. doi:10.1093/clinids/3.Supplement_2.S200. PMID 7043707.
- Orange Book: Approved Drug Products with Therapeutic Equivalence Evaluations Archived 2016-08-17 at the Wayback Machine
External links
- "Vancomycin". Drug Information Portal. U.S. National Library of Medicine.
- "Vancomycin hydrochloride". Drug Information Portal. U.S. National Library of Medicine.